Environmental toxicants, as detected by our TOXDetect Profile®, play a significant role in understanding our exposures. By recognizing how they are metabolized, even more insights can be gleaned about timing of exposure, the ability to eliminate them, and what additional influences they may be having on the system. It is essential to note that many of these toxicants are lipophilic, meaning they have an affinity for fat, allowing them to be more readily absorbed, particularly from dermal exposures.1 Being lipophilic means they also have a higher potential to be stored in lipids (e.g. cell membranes, adipose tissue), and need to go through biotransformation (i.e. Phase I and II of detoxification) to be eliminated.1 The chart below illustrates the parent compound, the metabolite of the parent compound, half-lives, and metabolic processes of these toxicants, including common classes such as phthalates, volatile organic compounds (VOCs), and pesticides. Phthalates, often found in plastics, VOCs from industrial emissions, and pesticides used in agriculture are prominent examples of environmental contaminants that the body must process and eliminate.
The liver is crucial in managing these lipophilic substances, as it transforms them through a series of detoxification phases to make them more hydrophilic (water-soluble) and easier to secrete. This process involves three key phases: Phase I, Phase II, and Phase III, each playing a unique role in detoxification. For a comprehensive understanding of these phases and nutrients that support these intrinsic reactions, you can refer to The Liver: It’s Important Role in Detoxification and The Liver: Supportive Nutrients in Detoxification. The first article details the phases and specific reactions involved in liver detoxification, while the second explores the nutrients and plant derivatives that enhance these processes and promote liver health.
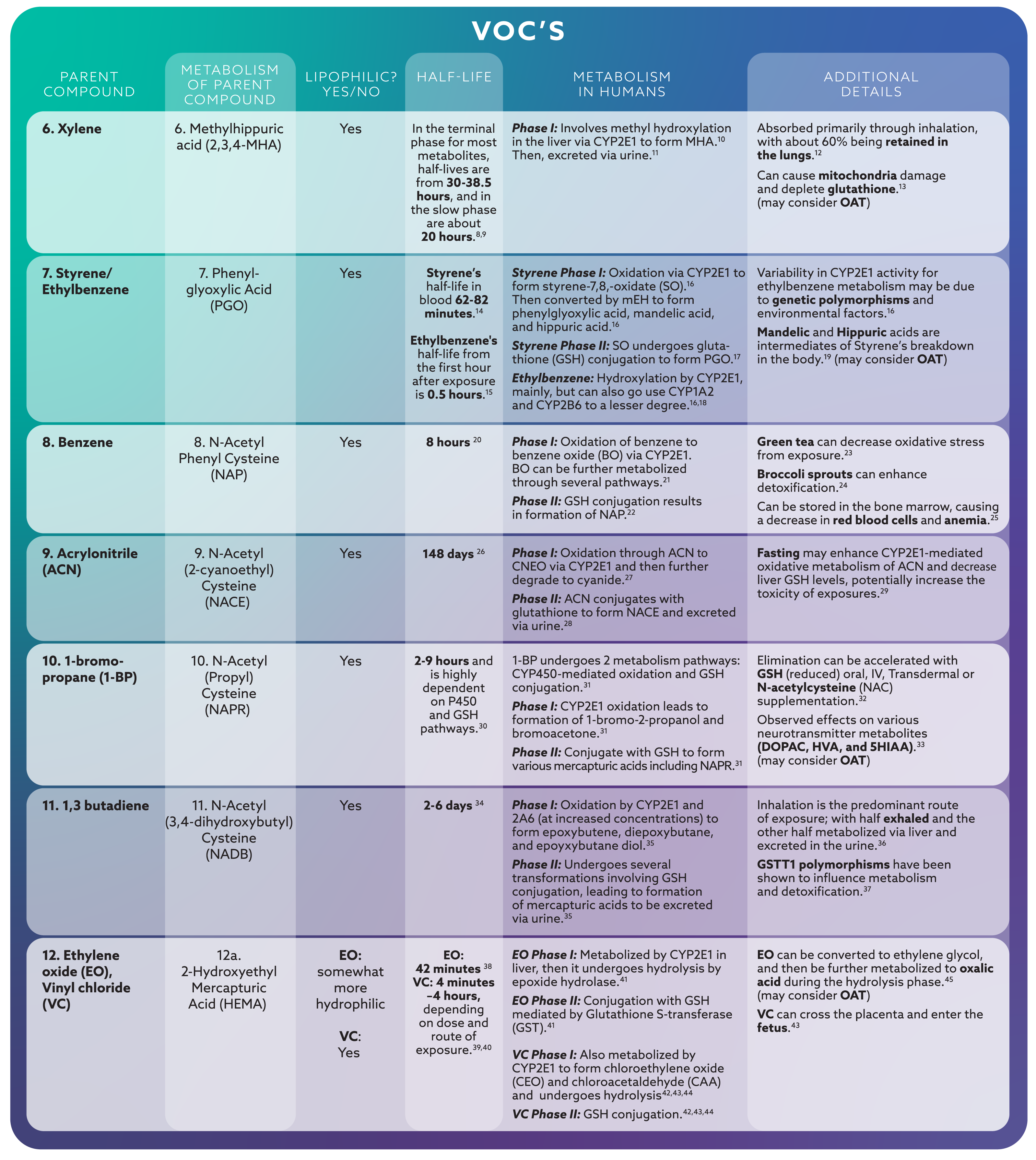
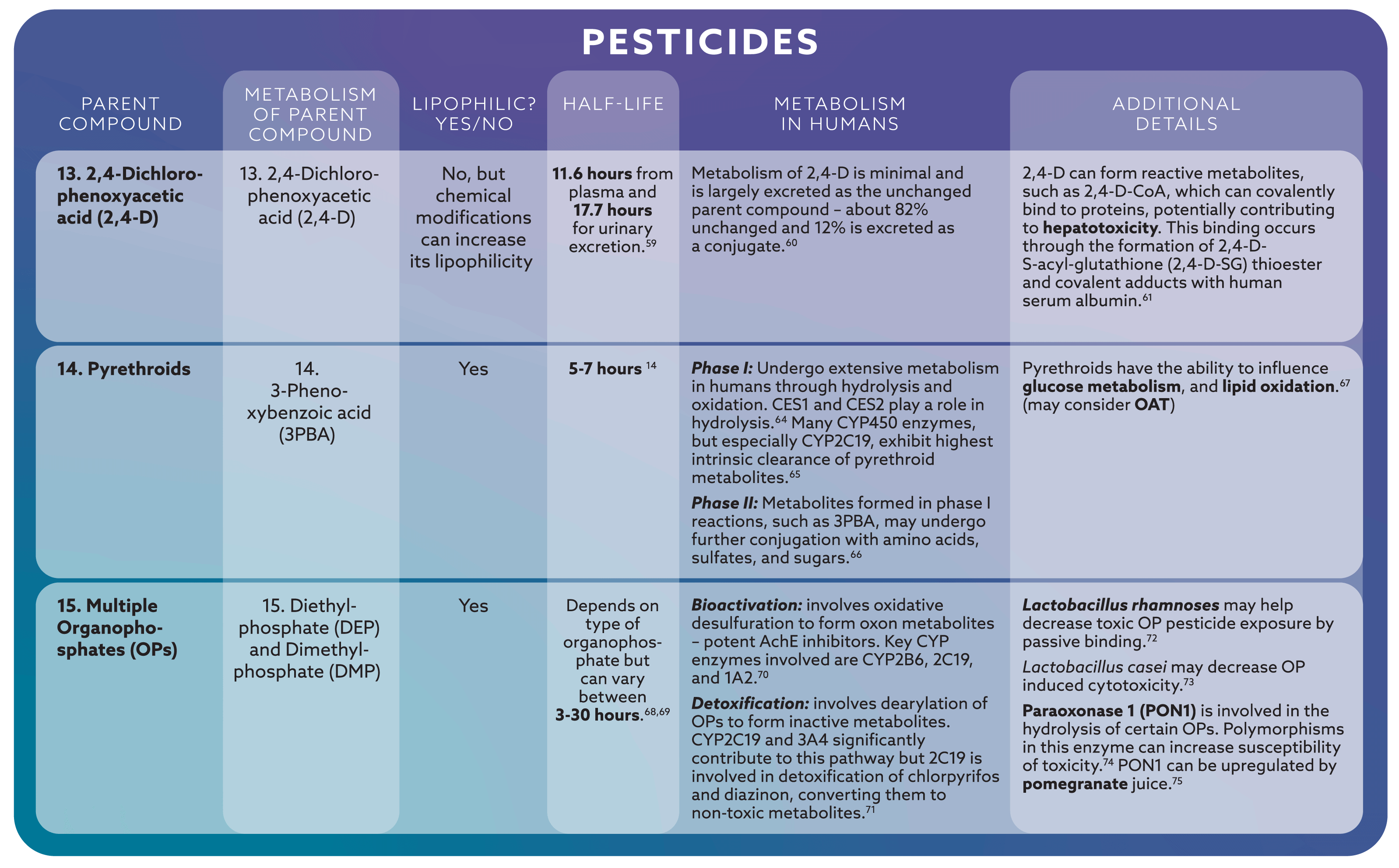
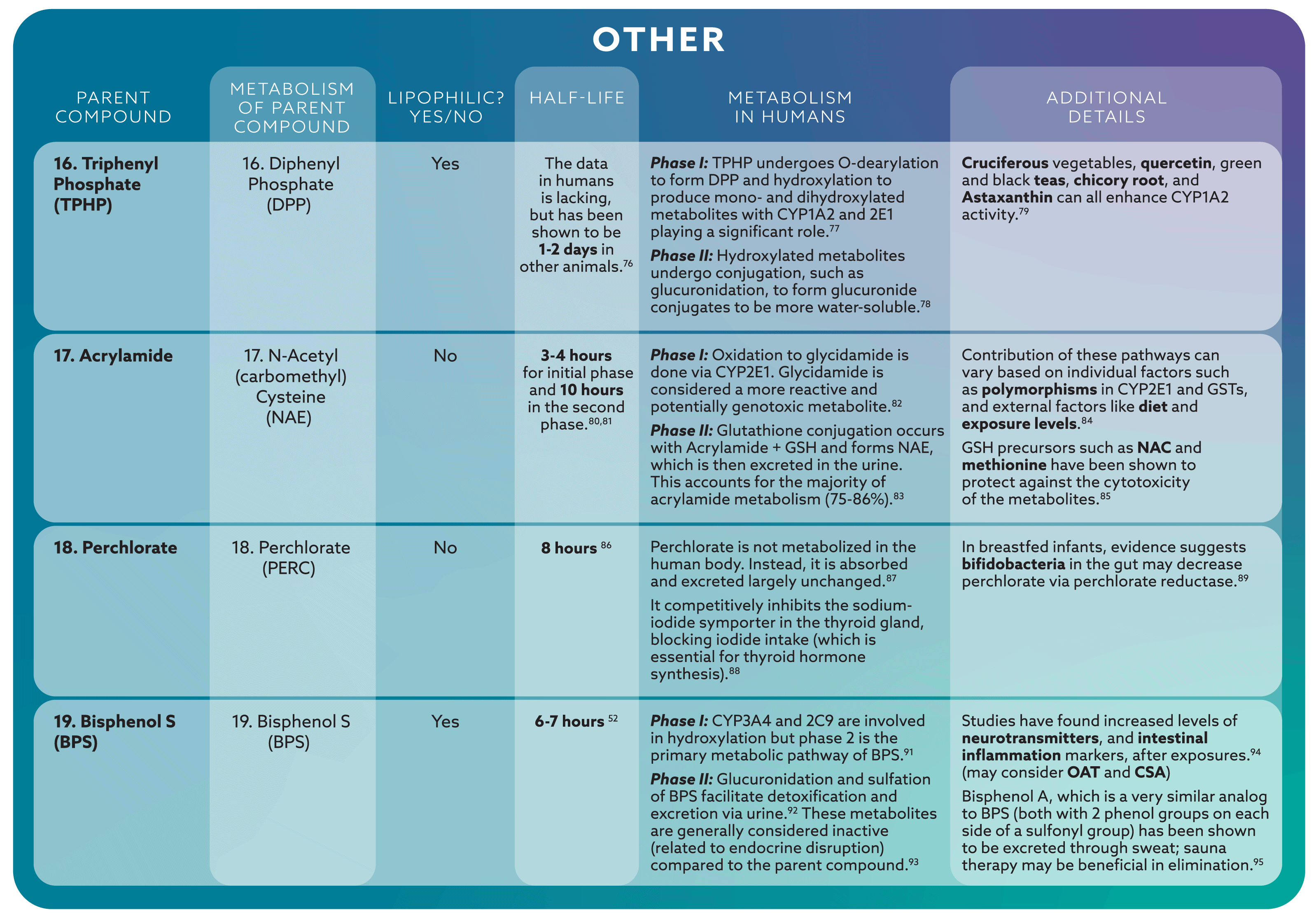
Summary
TOXDetect Profile® offers a comprehensive approach to evaluating environmental toxicant exposures, complemented by a thorough environmental exposure history intake. This combination equips practitioners with insights needed to understand how toxicants influence patient health and to implement evidence-based avoidance strategies, address health impacts, and tailor personalized therapeutic interventions. Our goal is to empower you to proactively mitigate the effects of environmental toxicants, fostering long-term health and well-being for both you and your patients.
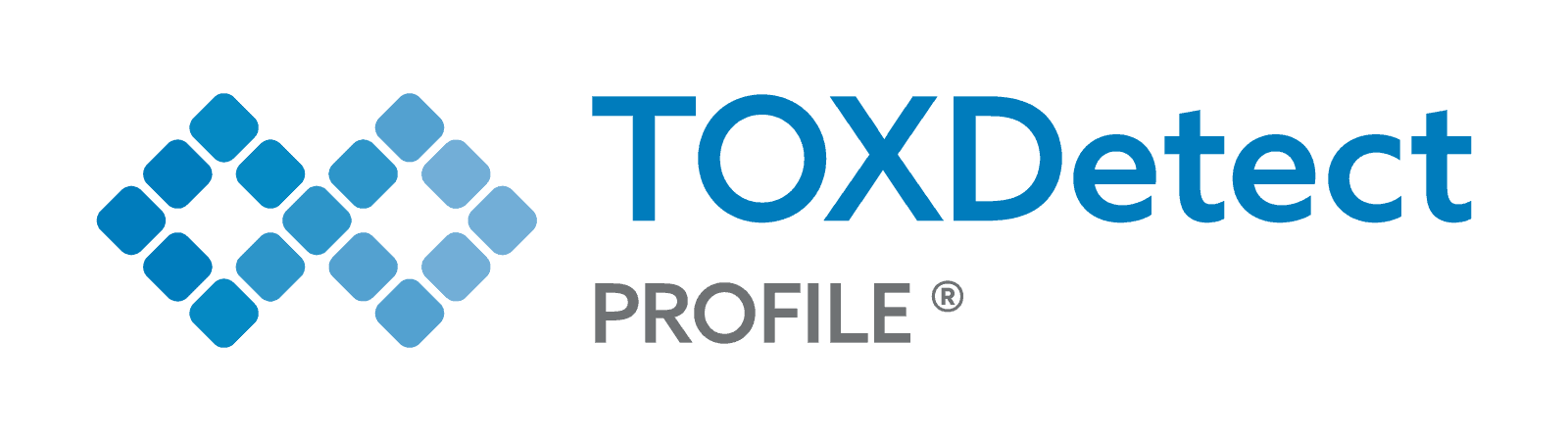
References
- Mittermeier A, Völkel W, Fromme H. Kinetics of the phthalate metabolites mono-2-ethylhexyl phthalate (MEHP) and mono-n-butyl phthalate (MnBP) in male subjects after a single oral dose. Toxicol Lett. 2016;252:22-28. doi:10.1016/j.toxlet.2016.04.009
- Humphrey KM, Pandey S, Martin J, Hagoel T, Grand’Maison A, Ohm JE. Establishing a role for environmental toxicant exposure induced epigenetic remodeling in malignant transformation. Semin Cancer Biol. 2019 Aug;57:86-94. doi: 10.1016/j.semcancer.2018.11.002. Epub 2018 Nov 16. PMID: 30453042
- In Vitro Metabolism of Di(2-Ethylhexyl) Phthalate (DEHP) by Various Tissues and Cytochrome P450s of Human and Rat. Choi K, Joo H, Campbell JL, et al. Toxicology in Vitro : An International Journal Published in Association With BIBRA. 2012;26(2):315-22. doi:10.1016/j.tiv.2011.12.002.
- Hydrolysis of Dibutyl Phthalate and Di(2-Ethylhexyl) Phthalate in Human Liver, Small Intestine, Kidney, and Lung: An in Vitro Analysis Using Organ Subcellular Fractions and Recombinant Carboxylesterases. Isobe T, Ohkawara S, Mori Y, et al.Chemico-Biological Interactions. 2023;372:110353. doi:10.1016/j.cbi.2023.110353.
- Genuis SJ, Beesoon S, Lobo RA, Birkholz D. Human elimination of phthalate compounds: blood, urine, and sweat (BUS) study. ScientificWorldJournal. 2012;2012:615068. doi:10.1100/2012/615068
- Kabekkodu, S. et al. The mitochondrial linkL Phthalate exposure and cardiovascular disease. BBA- Molecular Cell Research.
- Goyal, S. Et al. An insight into the critical role of tgut microbiota in triggering the phthalate-induced toxicity and it’s mitigation using probiotics. Science of the Total Environment. 2023. 904 (15); doi: 166889
- Adams JC, Dills RL, Morgan MS, Kalman DA, Pierce CH. A physiologically based toxicokinetic model of inhalation exposure to xylenes in Caucasian men. Regul Toxicol Pharmacol. 2005;43(2):203-214. doi:10.1016/j.yrtph.2005.07.005
- Riihimäki V, Pfäffli P, Savolainen K, Pekari K. Kinetics of m-xylene in man: General features of absorption, distribution, biotransformation and excretion in repetitive inhalation exposure. Scand J Work Environ Health. 1979;5(3):217-231. doi:10.5271/sjweh.3096
- Human Cytochrome P450 Isoform Specificity in the Regioselective Metabolism of Toluene and O-, M- And P-Xylene. The Journal of Pharmacology and Experimental Therapeutics. 1996;276(1):101-8. doi:10.1163/2211730x96x00063.
- Estimation of the Dermal Absorption of M-Xylene Vapor in Humans Using Breath Sampling and Physiologically Based Pharmacokinetic Analysis. Loizou GD, Jones K, Akrill P, Dyne D, Cocker J. Toxicological Sciences : An Official Journal of the Society of Toxicology. 1999;48(2):170-9. doi:10.1093/toxsci/48.2.170.
- Kinetics of M-Xylene in Man: General Features of Absorption, Distribution, Biotransformation and Excretion in Repetitive Inhalation Exposure. Riihimäki V, Pfäffli P, Savolainen K, Pekari K. Scandinavian Journal of Work, Environment & Health. 1979;5(3):217-31. doi:10.5271/sjweh.3096.
- Salimi A, Talatappe BS, et al. Xylene Induces Oxidative Stress and Mitochondria Damage in Isolated Human Lymphocytes. Toxicol Res. 2017 Jul;33(3):233-238. doi: 10.5487/TR.2017.33.3.233.
- Wenker MA, Kezić S, Monster AC, de Wolff FA. Stereochemical metabolism of styrene in volunteers. Int Arch Occup Environ Health. 2001;74(5):359-365. doi:10.1007/pl00007953
- Knecht U, Reske A, Woitowitz HJ. Biological monitoring of standardized exposure to ethylbenzene: evaluation of a biological tolerance (BAT) value. Arch Toxicol. 2000;73(12):632-640. doi:10.1007/s002040050018
- Investigation of Bioactivation and Toxicity of Styrene in CYP2E1 Transgenic Cells. Chung JK, Yuan W, Liu G, Zheng J. Toxicology. 2006;226(2-3):99-106. doi:10.1016/j.tox.2006.06.001.
- Atsdr.cdc.gov. 2022. [online] Available at: https://www.atsdr.cdc.gov/ToxProfiles/tp53-c1-b.pdf [Accessed 1 October 2022].
- Metabolism of Ethylbenzene by Human Liver Microsomes and Recombinant Human Cytochrome P450s (CYP). Sams C, Loizou GD, Cocker J, Lennard MS. Toxicology Letters. 2004;147(3):253-60. doi:10.1016/j.toxlet.2003.11.010.
- Qronfla MM,et. al. Synthesis and Characterization of a New Molecularly Imprinted Polymer for Selective Extraction of Mandelic Acid Metabolite from Human Urine as a Biomarker of Environmental and Occupational Exposures to Styrene. Polymers (Basel). 2023 May 21;15(10):2398. doi: 10.3390/polym15102398.
- Brugnone F, Perbellini L, Maranelli G, Romeo L, Guglielmi G, Lombardini F. Reference values for blood benzene in the occupationally unexposed general population. Int Arch Occup Environ Health. 1992;64(3):179-184. doi:10.1007/BF00380906
- The Fate of Benzene-Oxide. Monks TJ, Butterworth M, Lau SS. Chemico-Biological Interactions. 2010;184(1-2):201-6. doi:10.1016/j.cbi.2009.12.025.
- Ashraf M. Emara & Hoda El-Bahrawy (2008) Green Tea Attenuates Benzene-Induced Oxidative Stress in Pump Workers, Journal of Immunotoxicology, 5:1, 69- 80, DOI: 10.1080/15476910802019029
- Ashraf M. Emara & Hoda El-Bahrawy (2008) Green Tea Attenuates Benzene-Induced Oxidative Stress
- Chen JG, Johnson J, Egner P, et al. Dose-dependent detoxification of the airborne pollutant benzene in a randomized trial of broccoli sprout beverage in Qidong, China. Am J Clin Nutr. 2019;110(3):675-684. doi:10.1093/ajcn/nqz122
- Center for Disease Control. Facts about Benzene. 2018. https://emergency.cdc.gov/agent/benzene/basics/facts.asp#:~:text=Benzene%20causes%20harmful%20effects%20on,increasing%20the%20chance%20for%20infection.
- Bader M, Wrbitzky R. Follow-up biomonitoring after accidental exposure to acrylonitrile:- implications for protein adducts as a dose monitor for short-term exposures. Toxicol Lett. 2006;162(2-3):125-131. doi:10.1016/j.toxlet.2005.09.034
- Role of Cytochrome P450 2E1 in the Metabolism of Acrylamide and Acrylonitrile in Mice. Sumner SC, Fennell TR, Moore TA, et al. Chemical Research in Toxicology. 1999;12(11):1110-6. doi:10.1021/tx990040k.
- Species Differences in Acrylonitrile Metabolism and Toxicity Between Experimental Animals and Humans Based on Observations in Human Accidental Poisonings. Thier R, Lewalter J, Bolt HM. Archives of Toxicology. 2000;74(4-5):184-9. doi:10.1007/s002040000109
- Wang S, Xing G, Li F, et al. Fasting Enhances the Acute Toxicity of Acrylonitrile in Mice via Induction of CYP2E1. Toxics. 2022;10(6):337. Published 2022 Jun 19. doi:10.3390/toxics10060337
- Garner CE, Yu X. Species and sex-dependent toxicokinetics of 1-bromopropane: the role of hepatic cytochrome P450 oxidation and glutathione (GSH). Xenobiotica. 2014;44(7):644-656. doi:10.3109/00498254.2013.879624
- The Oxidative Metabolism of 1-Bromopropane in the Rat. Jones AR, Walsh DA. Xenobiotica; The Fate of Foreign Compounds in Biological Systems. 1979;9(12):763-72. doi:10.3109/00498257909042344.
- Species and Sex-Dependent Toxicokinetics of 1-Bromopropane: The Role of Hepatic Cytochrome P450 Oxidation and Glutathione (GSH). Garner CE, Yu X. Xenobiotica; The Fate of Foreign Compounds in Biological Systems. 2014;44(7):644-56. doi:10.3109/00498254.2013.879624.
- Center of Disease Control. ATSDR 1- Bromopropane Tox Profile. 2017. https://www.atsdr.cdc.gov/ToxProfiles/tp209.pdf
- Goggin M, Sangaraju D, Walker VE, Wickliffe J, Swenberg JA, Tretyakova N. Persistence and repair of bifunctional DNA adducts in tissues of laboratory animals exposed to 1,3-butadiene by inhalation. Chem Res Toxicol. 2011;24(6):809-817. doi:10.1021/tx200009b
- 1,3-Butadiene: I. Review of Metabolism and the Implications to Human Health Risk Assessment. Kirman CR, Albertini RJ, Sweeney LM, Gargas ML. Critical Reviews in Toxicology. 2010;40 Suppl 1:1-11. doi:10.3109/10408444.2010.507181.
- Atsdr.cdc.gov. 2022. 1,3-butadiene. [online] Available at: <https://www.atsdr.cdc.gov/toxprofiles/tp28-c3.pdf> [Accessed 1 October 2022].
- Boldry EJ, et, al. Genetic Determinants of 1,3-Butadiene Metabolism and Detoxification in Three Populations of Smokers with Different Risks of Lung Cancer. Cancer Epidemiol Biomarkers Prev. 2017 Jul;26(7):1034-1042. doi: 10.1158/1055-9965.EPI-16-0838.
- Filser JG, Denk B, Törnqvist M, Kessler W, Ehrenberg L. Pharmacokinetics of ethylene in man; body burden with ethylene oxide and hydroxyethylation of hemoglobin due to endogenous and environmental ethylene [published correction appears in Arch Toxicol 1993;67(3):230]. Arch Toxicol. 1992;66(3):157-163. doi:10.1007/BF01974008
- Zuccato E, Marcucci F, Fanelli R, Mussini E. Head-space gas-chromatographic analysis of vinyl chloride monomer in rat blood and tissues. Xenobiotica. 1979;9(1):27-31. doi:10.3109/00498257909034701
- Green T, Hathway DE. The biological fate in rats of vinyl chloride in relation to its oncogenicity. Chem Biol Interact. 1975;11(6):545-562. doi:10.1016/0009-2797(75)90030-7
- A Physiologically Based Toxicokinetic Model for Inhaled Ethylene and Ethylene Oxide in Mouse, Rat, and Human. Filser JG, Klein D. Toxicology Letters. 2018;286:54-79. doi:10.1016/j.toxlet.2017.07.896.
- Studies on the Metabolism of Vinyl Chloride. Antweiler H. Environmental Health Perspectives. 1976;17:217-9. doi:10.1289/ehp.7617217.
- ATSDR. ToxGuide for Vinyl Chloride. https://www.atsdr.cdc.gov/toxguides/toxguide-20.pdf
- Epa.gov. 2022. Acute exposure guideline levels for selected airborne chemicals. [online] Available at: <https://www.epa.gov/sites/default/files/2014-09/documents/ethyleneoxide_final_volume9_2010.pdf> [Accessed 1 October 2022].
- ATSDR. Ethylene Oxide: Chapter 3: Toxicokinetics, susceptible populations, biomarkers, and chemical interactions. https://www.atsdr.cdc.gov/ToxProfiles/tp137-c3.pdf
- Nihlén A, Löf A, Johanson G. Experimental exposure to methyl tertiary-butyl ether. I. Toxicokinetics in humans. Toxicol Appl Pharmacol. 1998;148(2):274-280. doi:10.1006/taap.1997.8333
- Amberg A, Rosner E, Dekant W. Biotransformation and kinetics of excretion of methyl-tert-butyl ether in rats and humans. Toxicol Sci. 1999;51(1):1-8. doi:10.1093/toxsci/51.1.1
- Metabolism of Methyl Tert-Butyl Ether and Other Gasoline Ethers by Human Liver Microsomes and Heterologously Expressed Human Cytochromes P450: Identification of CYP2A6 as a Major Catalyst. Hong JY, Wang YY, Bondoc FY, et al. Toxicology and Applied Pharmacology. 1999;160(1):43-8. doi:10.1006/taap.1999.8750.
- Atsdr.cdc.gov. 2022. Toxicological profile for MTBE. [online] Available at: <https://www.atsdr.cdc.gov/toxprofiles/tp91.pdf> [Accessed 1 October 2022].
- Biotransformation of 12c- And 2-13c-Labeled Methyl Tert-Butyl Ether, Ethyl Tert-Butyl Ether, and Tert-Butyl Alcohol in Rats: Identification of Metabolites in Urine by 13C Nuclear Magnetic Resonance and Gas Chromatography/Mass Spectrometry. Bernauer U, Amberg A, Scheutzow D, Dekant W. Chemical Research in Toxicology. 1998;11(6):651-8. doi:10.1021/tx970215v.
- Atsdr.cdc.gov. 2022. Toxicological profile for MTBE. [online] Available at: <https://www.atsdr.cdc.gov/toxprofiles/tp91.pdf> [Accessed 1 October 2022]
- Metabolism and Distribution of [2,3-14C]acrolein in Sprague-Dawley Rats. II. Identification of Urinary and Fecal Metabolites. Parent RA, Paust DE, Schrimpf MK, et al. Toxicological Sciences : An Official Journal of the Society of Toxicology. 1998;43(2):110-20. doi:10.1006/toxs.1998.2462
- Wang TW. Et al. Identification of acrolein metabolites in human buccal cells, blood, and urine after consumption of commercial fried foods. Food Science and Nutrition. 2019.;7:1668-1676. DOI: 10.1002/fsn3.1001
- Detoxication of the Environmental Pollutant Acrolein by a Rat Liver Aldo-Keto Reductase. Gardner R, Kazi S, Ellis EM. Toxicology Letters. 2004;148(1-2):65-72. doi:10.1016/j.toxlet.2003.12.056.
- Moghe A, et al. Molecular mechanisms of acrolein toxicity: relevance to human disease. Toxicol Sci. 2015 Feb;143(2):242-55. doi: 10.1093/toxsci/kfu233
- National Research Counsil (US) Committee on Acute Exposure Guideline levels. Acute Exposure Guideline Levels for Selected Airborne Chemicals Volume 9. Chapter 5: Propylene Oxide: Acute Exposure Guideline Levels. 2010. Propylene Oxide Acute Exposure Guideline Levels – Acute Exposure Guideline Levels for Selected Airborne Chemicals – NCBI Bookshelf (nih.gov)
- Kinetics of Propylene Oxide Metabolism in Microsomes and Cytosol of Different Organs From Mouse, Rat, and Humans. Faller TH, Csanády GA, Kreuzer PE, Baur CM, Filser JG. Toxicology and Applied Pharmacology. 2001;172(1):62-74. doi:10.1006/taap.2001.9135.
- Faller, T. H., Csanády, G. A., Kreuzer, P. E., Baur, C. M., & Filser, J. G. (2001). Kinetics of Propylene Oxide Metabolism in Microsomes
- Sauerhoff MW, Braun WH, Blau GE, Gehring PJ. The fate of 2,4-dichlorophenoxyacetic acid (2,4-D) following oral administration to man. Toxicology. 1977;8(1):3-11. doi:10.1016/0300-483x(77)90018-x
- The Fate of 2,4-Dichlorophenoxyacetic Acid (2,4-D) Following Oral Administration to Man. Sauerhoff MW, Braun WH, Blau GE, Gehring PJ. Toxicology. 1977;8(1):3-11. doi:10.1016/0300-483x(77)90018-x. and Cytosol of Different Organs from Mouse, Rat, and Humans. Toxicology and Applied Pharmacology, 172(1), 62–74. doi:10.1006/taap.2001.9135
- In Vitro Studies on the Chemical Reactivity of 2,4-Dichlorophenoxy Acetyl-S-Acyl-CoA Thioester. Li C, Grillo MP, Benet LZ. Toxicology and Applied Pharmacology. 2003;187(2):101-9. doi:10.1016/s0041-008x(02)00043-1.
- Leng G, Kühn KH, Idel H. Biological monitoring of pyrethroids in blood and pyrethroid metabolites in urine: applications and limitations. Sci Total Environ. 1997;199(1-2):173-181. doi:10.1016/s0048-9697(97)05493-4
- Starr JM, Graham SE, Ross DG, et al. Environmentally relevant mixing ratios in cumulative assessments: a study of the kinetics of pyrethroids and their ester cleavage metabolites in blood and brain; and the effect of a pyrethroid mixture on the motor activity of rats. Toxicology. 2014;320:15-24. doi:10.1016/j.tox.2014.02.016
- Biotransformation and Enzymatic Reactions of Synthetic Pyrethroids in Mammals. Mikata K, Isobe N, Kaneko H. Topics in Current Chemistry. 2012;314:113-35. doi:10.1007/128_2011_254.
- Metabolism of Deltamethrin and Cis- And Trans-Permethrin by Human Expressed Cytochrome P450 and Carboxylesterase Enzymes. Hedges L, Brown S, MacLeod AK, et al. Xenobiotica; The Fate of Foreign Compounds in Biological Systems. 2019;49(5):521-527. doi:10.1080/00498254.2018.1474283.
- The Pyrethroid Metabolites 3-Phenoxybenzoic Acid and 3-Phenoxybenzyl Alcohol Do Not Exhibit Estrogenic Activity in the McF-7 Human Breast Carcinoma Cell Line or Sprague-Dawley Rats. Laffin B, Chavez M, Pine M. Toxicology. 2010;267(1-3):39-44. doi:10.1016/j.tox.2009.10.003
- Hodoșan C, Gîrd CE, Ghica MV, Dinu-Pîrvu CE, Nistor L, Bărbuică IS, Marin ȘC, Mihalache A, Popa L. Pyrethrins and Pyrethroids: A Comprehensive Review of Natural Occurring Compounds and Their Synthetic Derivatives. Plants (Basel). 2023 Nov 29;12(23):4022. doi: 10.3390/plants12234022
- Hoffmann U, Papendorf T. Organophosphate poisonings with parathion and dimethoate. Intensive Care Med. 2006;32(3):464-468. doi:10.1007/s00134-005-0051-z
- Jose A, Selvakumar R, Peter JV, Karthik G, Fleming DH, Fleming JJ. Estimation of Monocrotophos renal elimination half-life in humans. Clin Toxicol (Phila). 2015;53(7):629-632. doi:10.3109/15563650.2015.1054500
- Human Hepatic Cytochrome P450-Specific Metabolism of the Organophosphorus Pesticides Methyl Parathion and Diazinon. Ellison CA, Tian Y, Knaak JB, Kostyniak PJ, Olson JR. Drug Metabolism and Disposition: The Biological Fate of Chemicals. 2012;40(1):1-5. doi:10.1124/dmd.111.042572.
- Metabolism of Chlorpyrifos by Human Cytochrome P450 Isoforms and Human, Mouse, an
- Trinder M, McDowell TW, Daisley BA, et al. Probiotic Lactobacillus rhamnosus Reduces OrganophosphatePesticide Absorption and Toxicity to Drosophila melanogaster. Appl Environ Microbiol. 2016;82(20):6204-6213. Published 2016 Sep 30. doi:10.1128/AEM.01510-16 d Rat Liver Microsomes. Tang J, Cao Y, Rose RL, et al. Drug Metabolism and Disposition: The Biological Fate of Chemicals. 2001;29(9):1201-4.
- Bagherpour Shamloo H, Golkari S, Faghfoori Z, et al. Lactobacillus Casei Decreases Organophosphorus Pesticide Diazinon Cytotoxicity in Human HUVEC Cell Line. Adv Pharm Bull. 2016;6(2):201-210. doi:10.15171/apb.2016.028
- Costa LG, et al. Paraoxonase 1 (PON1) as a genetic determinant of susceptibility to organophosphate toxicity. Toxicology. 2013 May 10;307:115-22. doi: 10.1016/j.tox.2012.07.011.
- Parsaeyan N, et al. Effect of pomegranate juice on paraoxonase enzyme activity in patients with type 2 diabetes. J Diabetes Metab Disord. 2012 Aug 31;11(1):11. doi: 10.1186/2251-6581-11-11.
- Marteinson S, Guigueno MF, Fernie KJ, Head JA, Chu S, Letcher RJ. Uptake, Deposition, and Metabolism of Triphenyl Phosphate in Embryonated Eggs and Chicks of Japanese Quail (Coturnix japonica). Environ Toxicol Chem. 2020;39(3):565-573. doi:10.1002/etc.4637
- Metabolic Mechanism of Aryl Phosphorus Flame Retardants by Cytochromes P450: A Combined Experimental and Computational Study on Triphenyl Phosphate. Zhang Q, Ji S, Chai L, et al. Environmental Science & Technology. 2018;52(24):14411-14421. doi:10.1021/acs.est.8b03965.
- Determination of Glucuronide Conjugates of Hydroxyl Triphenyl Phosphate (OH-TPHP) Metabolites in Human Urine and Its Use as a Biomarker of TPHP Exposure. Su G, Letcher RJ, Yu H, Gooden DM, Stapleton HM. Chemosphere. 2016;149:314-9. doi:10.1016/j.chemosphere.2016.01.114.
- Hodges RE, Minich DM. Modulation of Metabolic Detoxification Pathways Using Foods and Food-Derived Components: A Scientific Review with Clinical Application. J Nutr Metab. 2015;2015:760689. doi: 10.1155/2015/760689. Epub 2015 Jun 16. PMID: 26167297; PMCID: PMC4488002.
- Fennell TR, Sumner SC, Snyder RW, Burgess J, Friedman MA. Kinetics of elimination of urinary metabolites of acrylamide in humans. Toxicol Sci. 2006;93(2):256-267. doi:10.1093/toxsci/kfl069
- Boettcher MI, Bolt HM, Drexler H, Angerer J. Excretion of mercapturic acids of acrylamide and glycidamide in human urine after single oral administration of deuterium-labelled acrylamide. Arch Toxicol. 2006;80(2):55- 61. doi:10.1007/s00204-005-0011-y
- In Vivo Role of Cytochrome P450 2E1 and Glutathione-S-Transferase Activity for Acrylamide Toxicokinetics in Humans. Doroshyenko O, Fuhr U, Kunz D, et al. Cancer Epidemiology, Biomarkers & Prevention : A Publication of the American Association for Cancer Research, Cosponsored by the American Society of Preventive Oncology. 2009;18(2):433-43. doi:10.1158/1055-9965.EPI-08-0832.
- Comparison of Acrylamide Metabolism in Humans and Rodents. Fennell TR, Friedman MA. Advances in Experimental Medicine and Biology. 2005;561:109-16. doi:10.1007/0-387-24980-X_9.
- Excretion of Mercapturic Acids of Acrylamide and Glycidamide in Human Urine After Single Oral Administration of Deuterium-Labelled Acrylamide. Boettcher MI, Bolt HM, Drexler H, Angerer J. Archives of Toxicology. 2006;80(2):55-61. doi:10.1007/s00204-005-0011-y.
- Kurebayashi H, Ohno Y. Metabolism of acrylamide to glycidamide and their cytotoxicity in isolated rat hepatocytes: protective effects of GSH precursors. Arch Toxicol. 2006;80(12):820-828. doi:10.1007/s00204-006-0109-x
- Pleus RC, Corey LM. Environmental exposure to perchlorate: A review of toxicology and human health. Toxicol Appl Pharmacol. 2018;358:102-109. doi:10.1016/j.taap.2018.09.001
- Perchlorate Clinical Pharmacology and Human Health: A Review. Soldin OP, Braverman LE, Lamm SH. Therapeutic Drug Monitoring. 2001;23(4):316-31. doi:10.1097/00007691-200108000-00002
- Perchlorate and the Thyroid Gland. Wolff J. Pharmacological Reviews. 1998;50(1):89-105.
- Breastfed Infants Metabolize Perchlorate. Shelor CP, Kirk AB, Dasgupta PK, et al. Environmental Science & Technology. 2012;46(9):5151-9. doi:10.1021/es2042806.
- Oh J, Choi JW, Ahn YA, Kim S. Pharmacokinetics of bisphenol S in humans after single oral administration. Environ Int. 2018;112:127-133. doi:10.1016/j.envint.2017.11.020
- Influence of Metabolism on Endocrine Activities of Bisphenol S. Skledar DG, Schmidt J, Fic A, et al. Chemosphere. 2016;157:152-9. doi:10.1016/j.chemosphere.2016.05.027.
- Last Piece in the Puzzle of Bisphenols BPA, BPS and BPF Metabolism: Kinetics of the in Vitro Sulfation Reaction. Durcik M, Gramec Skledar D, Tomašič T, Trontelj J, Peterlin Mašič L. Chemosphere. 2022;303(Pt 2):135133. doi:10.1016/j.chemosphere.2022.135133.
- Disposition and Metabolism of the Bisphenol Analogue, Bisphenol S, in Harlan Sprague Dawley Rats and B6c3f1/N Mice and in Vitro in Hepatocytes From Rats, Mice, and Humans. Waidyanatha S, Black SR, Snyder RW, et al. Toxicology and Applied Pharmacology. 2018;351:32-45. doi:10.1016/j.taap.2018.05.008.
- Junjie A. et al. Bisphenol S exposure induces intestinal inflammation: An integrated metabolomic and transcriptomic study,
- Chemosphere. Volume 292. 2022.133510. https://doi.org/10.1016/j.chemosphere.2021.133510