ABSTRACT: Context-Certain compounds found by gas chromatography-mass spectrometry in urine samples of children with autism might be produced by yeast in the gastrointestinal tract. Therefore, treatment with antifungal drugs might reduce clinical symptoms of autism.
Objective
To determine if symptoms of autism and chemical compounds in urine samples of children with autism decreased after antifungal treatment.
Design
A number of urinary organic acids first characterized as elevated in 2 brothers with autism were tested in urine samples of 23 children with autism (21 boys and 2 girls) before and after treatment with the antifungal drug nystatin.
Patients
Twenty-one boys and 2 girls with a mean age of 7.1 years with diagnosis of autism. Twenty boys and 17 girls with a mean age of 7.7 years, who were normal children of hospital employees, served as controls for urine testing.
Interventions
Children with abnormal baseline organic acid results received oral nystatin suspension at a dose of 100,000 units 4 times a day for 10 days. If abnormalities were still present after 10 days, an additional 60 days of antifungal treatment were offered. An additional urine organic acid test was then performed.
Results
The mean childhood autism rating scale, an indicator of the severity of autism, improved significantly after antifungal treatment based on the paired t test (P=.037). Seven of the urine markers were significantly higher in autistic males than in the control males. The concentration of several of the urine markers of autistic children decreased after antifungal treatment.
Conclusions
Antifungal drug therapy may be a promising therapeutic method for the treatment of autism. Organic acid testing appears to be a useful diagnostic test to indicate an overgrowth of yeast and bacteria in the gastrointestinal tract of children with autism and to predict the response of antifungal drug therapy, and may indicate relapse following the cessation of antifungal drug therapy. (Clinical Practice of Alternative Medicine 1(1): 15-26, 2000)
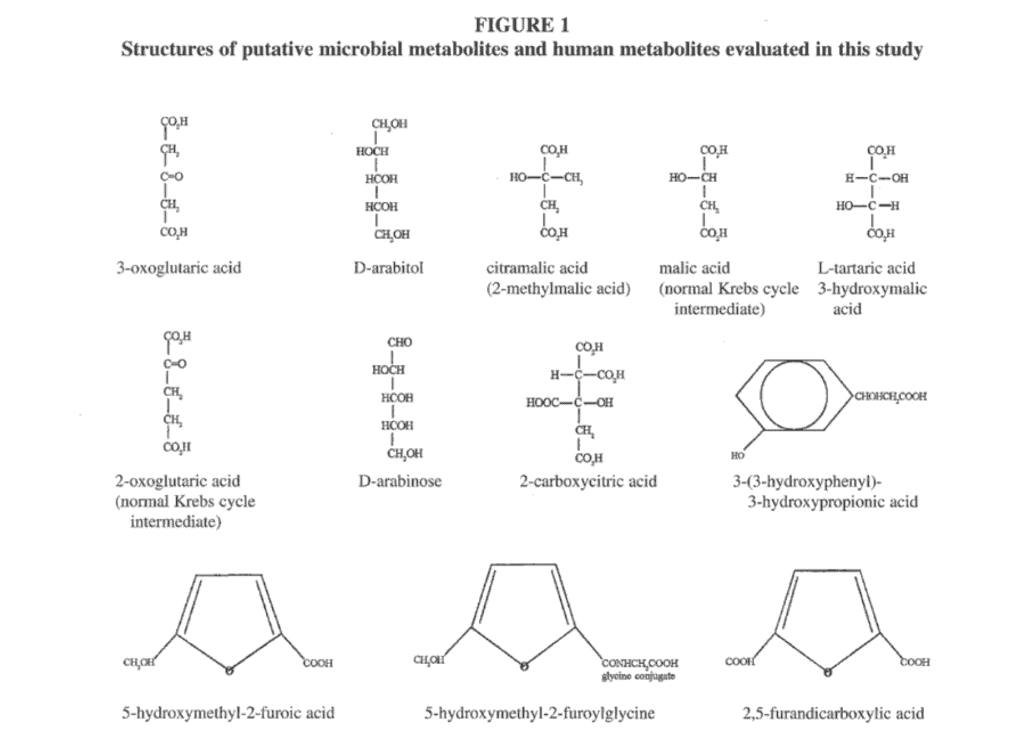
Our interest in a possible metabolic cause for autism was stimulated by the referral of 2 brothers with autism, on whom numerous urinary organic acid profiles were performed over a 2-year period.1 These tests revealed a consistent excretion of a number of compounds of possible microbial origin identified as the carbohydrate arabinose, analogs of normal Krebs cycle intermediates including 3-oxoglutaric acid, tartaric (3-hydroxymalic) acid, citramalic (methylmalic) acid, and a new tentatively identified analog of citric acid (carboxycitric acid) not previously reported in the medical literature.1 High concentrations of a compound identified as a phenylcarboxylic acid were also found; the mass spectrum of this compound did not correspond to the mass spectrum of any known compound in gas chromatography/mass spectrometry (GC/MS) libraries.1 Hydroxymethylfurancarboxylic acid, furandicarboxylic acid, furancarbonylglycine, and 3-(3-hydroxyphenyl)-3-hydroxypropionic acid were also found in urine samples of the 2 autistic brothers. Elevations of the latter 4 compounds were not previously reported by Shaw et al1; each of these compounds was positively identified by comparison to mass spectra in GC/MS libraries, published spectra, or by mass spectra provided by other GC/MS laboratories. The structures of the above compounds and closely related compounds are shown in Figure 1.
These findings of chemicals of possible microbial origin are of particular interest because of a report that autistic children have a greater incidence of ear infections than peers of the same age; that lower functioning autistic children had an earlier onset of ear infections than their higher functioning autistic peers; and that the ears of children with autism were anatomically positioned differently than those of normal children, perhaps leading to greater ear infection susceptibility.2 The use of oral antibiotics is the most prevalent therapy for ear infections in the US and many other nations. Furthermore, yeast and pathogenic bacterial overgrowth of the gastrointestinal (GI) tract commonly follows the use of oral antibiotics.3-15 Therefore, compounds produced by antibiotic-resistant bacteria, yeast, or fungi in the GI tract and then absorbed into the bloodstream might be involved in the etiology of autism just as abnormal elevations of phenylalanine and its metabolites cause the disease phenylketonuria (PKU). The production of microbial compounds due to microbial overgrowth of the GI tract would be acquired rather than genetic, although genetic susceptibility could be important in both reduced immunity or reduced ability to detoxify microbial products.
A review of the medical records from a group of autistic patients at the outpatient clinic of the pediatric hospital where this study was performed revealed a high frequency of multiple ear infections or sore throats treated with multiple courses of broad-spectrum antibiotics in infancy. Thrush in infancy was also common. A 2-year-old, developmentally normal child had a rapid onset of autistic symptoms that coincided with Candidiasis following multiple courses of antibiotic therapy for otitis media. This child excreted large quantities of tartaric acid, which decreased with nystatin therapy concomitant with improved clinical symptoms such as improved eye contact, decreased hyperactivity, and increased vocalization.
Based on these initial results, we decided to undertake a formal evaluation of the possible relationship between these elevated metabolites, possible yeast overgrowth, and autism. The objectives of the study were (1) to determine if the urinary excretion of abnormal Krebs cycle metabolites, abnormal carbohydrates, and other compounds of possible microbial origin are biochemical characteristics in autistic children, and (2) to determine if antifungal treatment results in decrease or elimination of abnormal metabolites in autistic children and/or improvement in autistic symptoms.
Methods and Materials
The proposed study comprised 23 autistic children, including the 2 siblings previously reported,1 from the outpatient department of a pediatric hospital. The study was reviewed and approved by the Institutional Review Board. Each child was examined by a pediatric neurologist, and then classified as autistic according to the latest criteria proposed by the American Psychiatric Association’s Diagnostic Statistical Manual of Mental Disorders.16 After informed consent, a random urine sample was collected without special preparation for organic acid analysis by GC/MS for the presence of abnormal metabolites. A solvent extract of the urine sample was derivatized by trimethylsilylation and then analyzed with a GC/MS, as described by Shaw et al.1 No analytical standards were available for the furan compounds, and quantification of these compounds was performed by assigning the response of an average size ion chromatogram peak as 100 units and then calibrating all other peaks of the same compounds against these arbitrary calibrators; each of these compounds was positively identified by comparison to mass spectra in a GC/MS library. For quantitative analysis of 3-(3-hydroxyphenyl)-3-hydroxypropionic acid, 3,4 -dihydroxyphenylpropionic acid was used as a surrogate calibration standard since 3-(3- hydroxyphenyl)-3-hydroxypropionic acid is not commercially available. The reconstructed ion chromatograph signal of the ion at mass to charge ratio 398 was used for quantitation. Similar use of surrogate standards is used extensively in the GC/MS analysis of environmental compounds in methods approved by the United States Environmental Protection Agency.
If the presence of abnormal metabolites was detected in the baseline urine sample (the sample collected before antifungal therapy), the child was offered treatment of suspected yeast infection with nystatin 100,000 units 4 times daily orally for 10 days. Then, another random urine sample was obtained and analyzed for organic acids. If the presence of abnormal metabolites was detected in the second urine sample, the child was offered a second course of treatment with nystatin for 2 months, and another urine sample was tested.
The patients’ baseline urine values served as controls. Urine samples from normal children of hospital employees served as additional controls. All of the control children were in good health and had no significant diseases or developmental disorders. An assessment of the severity of autistic behaviors was done by both a staff psychologist working with parents and by teachers using the Childhood Autism Rating Scale (CARS) scale.17 This assessment was done both at the beginning and at the end of nystatin therapy. The use of 2 evaluators was included to increase the reliability of this test. Furthermore, parents and teachers observed the children for extensive periods of time.
All 23 children were evaluated and their baseline urine was evaluated. One of the families moved shortly after the study began and was lost to follow-up. Thus, only 22 of 23 children completed the first course of nystatin therapy. Seven children did not complete the second course of nystatin for a variety of reasons. One of the children was dropped from the study after the first nystatin trial because the parent feared that the nystatin might exacerbate a preexisting colitis condition for which the child was hospitalized. One of the children refused to take the drug. In another case, the personnel at a child’s school were uncooperative in administering the medication.
Statistical analysis of the data was done as follows: The concentrations of each organic acid or other compounds in the groups of male or female children with autism were compared to the control groups using t tests that assumed unequal variances. For comparison of patients following treatment with nystatin, or after completion of therapy, paired t tests were performed on each metabolite concentration so that the value of each metabolite in the treatment groups was paired with the same patient’s baseline value. This statistical technique is sensitive in detecting small differences due to treatment.
Results
Characteristics of Patient and Control Populations
The patients who were initially recruited included 21 males and 2 females. None of these children had organic acid abnormalities characteristic of any of the well defined inborn errors of metabolism based on baseline organic acid testing. Patients were admitted into the study in the order in which the parents or guardians applied. The sex distribution of the controls included 17 females and 20 males. The mean age of the autistic children at the time of admission into the study was 7.1 years (SD=3.2), with a median age of 7.4 years and a range of 2.4 to 12.7 years. The mean age of the normal children at the time of urine collection was 7.7 years (SD=3.0), with a median age of 7.7 years and a range of 3.1 to 12.9 years. The mean age of the male autistic children at the time of admission into the study was 7.1 years (SD=3.3), with a median age of 7.4 years and a range of 2.4 to 12.7 years. The mean age of the male normal children at the time of urine collection was 8.1 years (SD=2.7), with a median age of 8.2 years and a range of 3.3 to 12.8 years. The mean age of the female autistic children at the time of admission into the study was 6.7 years (SD=2.1), with a median age of 6.7 years and a range of 5.2 to 8.2 years. The mean age of the female normal children at the time of urine collection was 7.8 years (SD=3.3), with a median age of 7.3 years and a range of 3.3 to 12.9 years.
Differences in Concentrations of Metabolites in Normal and Autistic Children
Because of the high percentage of autistic males entered into the study (91.7%), comparisons of the urine metabolite concentrations in autistic children were made only to controls of the same sex. Comparison of the urine metabolite concentrations of autistic males and control males (Table 1) revealed that the mean values for all of the urine metabolite concentrations except carboxycitric acid were higher in the autistic group than in the control group. Eight of these 9 mean values of the autistic males were significantly higher than those of the control males (P<.05). The median values of the autistic males for 8 of the 10 metabolites were also higher in the autistic male group. The mean difference between the autistic and normal males was greater than 60% for all of the metabolites except 3-(3-hydroxyphenyl)-3- hydroxypropionic acid and carboxycitric acid. The mean arabinose concentration of the autistic males was 5 times greater than that of the control males, and the median value for the arabinose concentration was 6 times that of the control males. The median value for urinary tartaric acid concentration in the autistic males was over 6 times that of the control males, but the t test significance was borderline (P=.097). The limited data for the autistic females (n = 2) restricted any generalizations about this group compared to normal females.
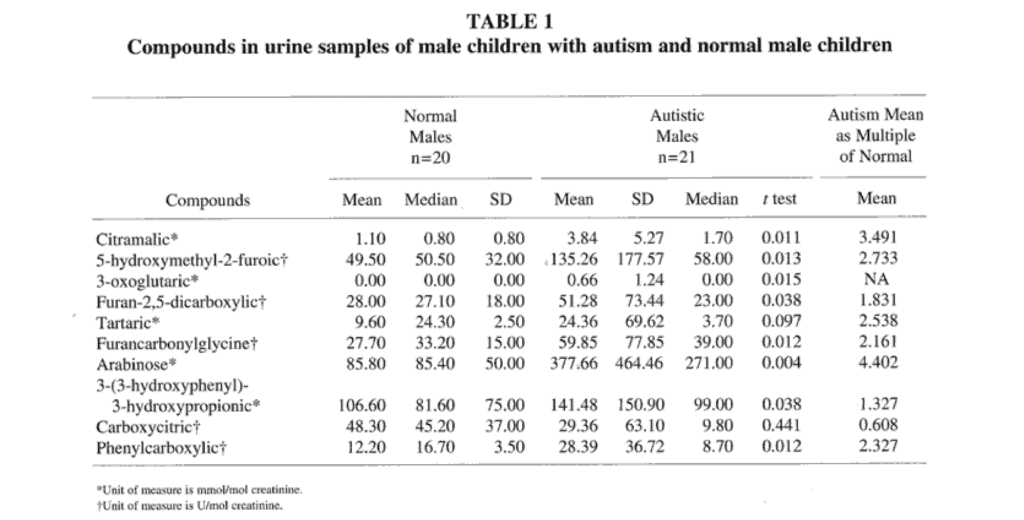
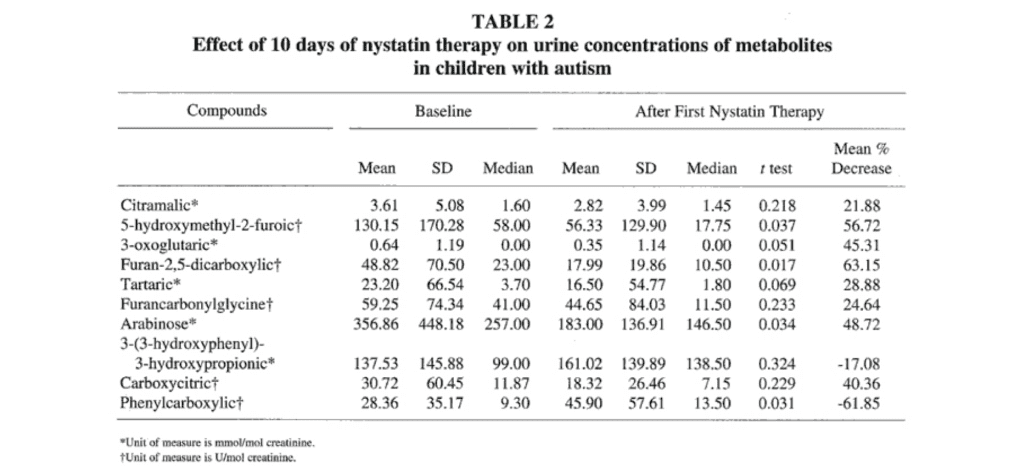
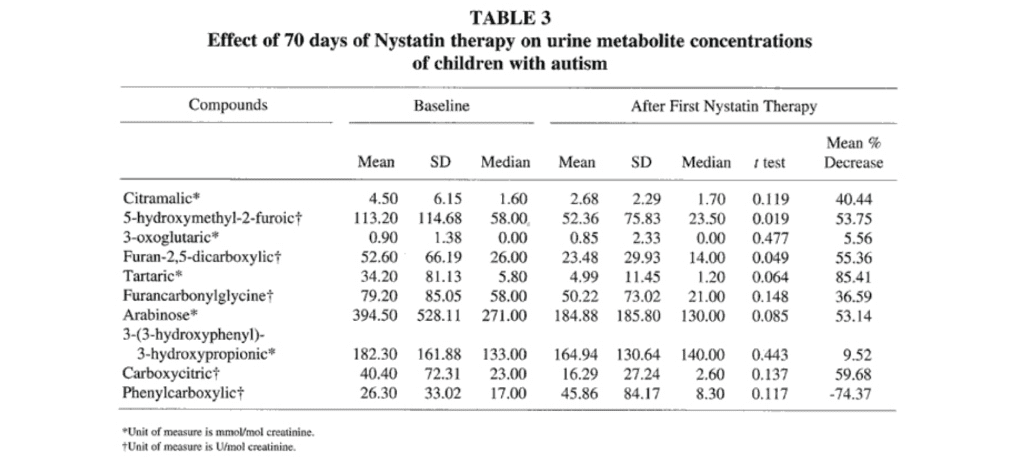
Effect of Antifungal Therapy on Concentrations of Metabolites
First Course of Nystatin Therapy. Of the 23 autistic children admitted into the antifungal drug study, 22 completed nystatin therapy and had their urine retested following this therapy. Eight of the 10 mean values for the urine metabolite concentrations decreased from baseline values following the first 10 days of nystatin therapy (Table 2). The mean concentrations of 5- hydroxymethyl 2-furoic acid, furan-2,5-dicarboxylic acid, and arabinose decreased significantly (P<.05). The mean values for the urine metabolite concentrations of phenylcarboxylic acid and 3-(3- hydroxyphenyl)-3-hydroxypropionic acid increased from baseline values following nystatin therapy; the mean concentration of phenylcarboxylic acid increased by 61.9% (P=.031).
Second Course of Nystatin Therapy. Fifteen of the 23 subjects admitted into the antifungal drug study completed the second course of nystatin therapy (an additional 60 days), and their urine was retested following this therapy. Nine of the 10 mean values for the urine metabolite concentrations decreased from baseline values following the second course of nystatin therapy (Table 3). Baseline values in Table 3 are different from those in Table 2 because only baseline values for those completing the second course of nystatin were included in Table 3. The mean concentrations of 5-hydroxymethyl-2-furoic acid and furan-2,5-dicarboxylic acid decreased significantly (P<.05), but the significance for the arabinose decrease was P=.085. The mean value for the urine concentration of the phenylcarboxylic acid compound increased 74.4 % (P=.117) from baseline values following the second course of nystatin therapy.
The response to nystatin is well-illustrated (Figure 2) in a child that had the highest baseline excretion of tartaric acid (300 mmol/mol creatinine), an abnormal value that was about 20 times the median normal value. Following the treatment with the nystatin, the tartaric acid continued to decrease. After 68 days, the parent began administering only 50% of the dose to avoid running out of nystatin completely. During that time, the tartaric acid began to increase. When the nystatin prescription was refilled and full doses of nystatin were administered, the tartaric acid again decreased.
CARS Evaluations. The CARS evaluations were completed for 18 of the children, 16 males and 2 females. The key to the CARS score scale is as follows: 15 to 30.0, normal; 30.1 to 37.0, mild to moderate autism; 37.1 to 60, severe autism. The baseline mean and median scores were 39.07 and 38.00, respectively, indicating that the group as a whole was in the severe autism range. The range in baseline scores is 30.5 to 53.5.
Eleven of the children were scored in the severe autism range, whereas 7 were scored in the mild to moderate range. Nine of the 18 children were reevaluated after the completion of nystatin therapy; the mean and median scores were 34.57 and 32.50, respectively. The mean decrease in the CARS score was statistically significant (P=.037) using the paired test. Improvements in the clinical symptoms of autism that were cited by the parents and teachers of the autistic children included decreased hyperactivity, increased eye contact and vocalization, better sleep patterns and concentration, increased imaginative play, reduced stereotypical behaviors such as spinning objects, and better academic performance. Several parents reported a loss of gains made by their child during antifungal drug therapy when the antifungal drug therapy was stopped and elected to reinstate the antifungal drug therapy. Some of the children have stayed on this therapy for more than 2 years and the parents report continued benefits to their child.
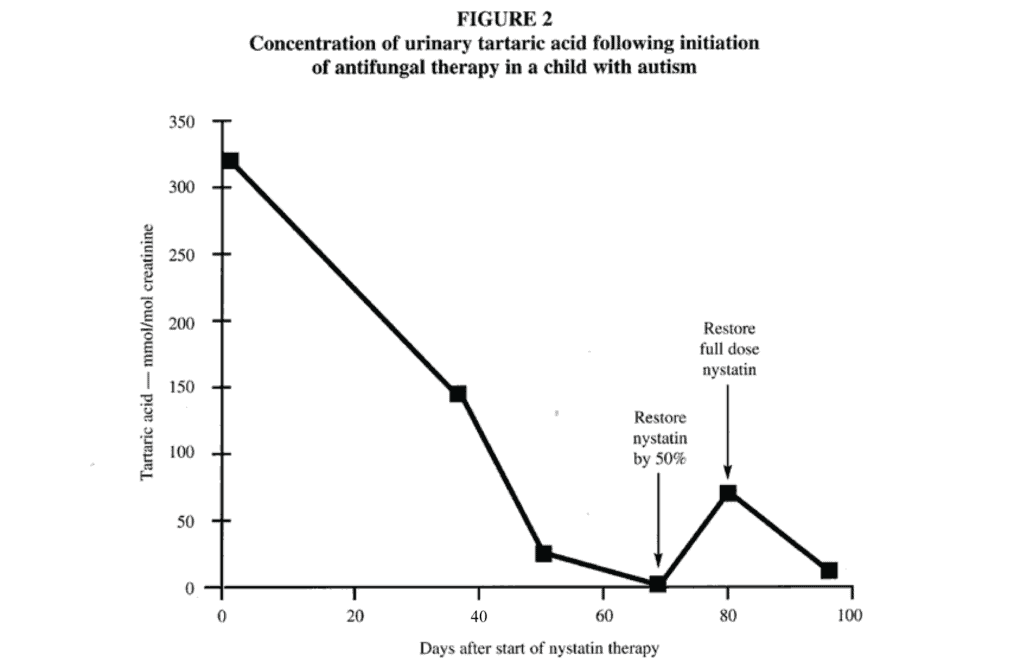
Discussion
Based on the reduction of the concentration of these compounds in urine after antifungal therapy, it seems likely that the furan compounds and arabinose are yeast or fungal metabolites and that tartaric, citramalic, and carboxycitric acids are metabolites of yeast or fungi.
Two of the compounds, 3-(3-hydroxyphenyl)-3-hydroxypropionic acid and the partially identified phenylcarboxylic acid that was commonly elevated in the urine of children with autism, were initially suspected of being yeast metabolites. However, these 2 compounds increased in the urine samples of children with autism after antifungal drug therapy. Later research established that these compounds were phenylalanine metabolites of several clostridia species (W. Shaw, unpublished data, 1999), which may also proliferate in the GI tract after most of the common broad-spectrum oral antibiotics.11-15 Berg13 reported that oral antibiotic treatment disrupts the normal ecology of the GI tract, allowing certain antibiotic-resistant indigenous bacteria to overpopulate while there is as much as a thousand fold decrease in the total number of anaerobic bacteria; following this overpopulation, these bacteria translocate from the GI tract to the mesenteric lymph nodes and possibly to other organs. Organisms of the Clostridium family, such as C botulinum, C. tetani, and C perfringens, are resistant to most of the broad-spectrum antibiotics commonly used to treat otitis media.
Yeast cultures on blood were not included on the protocol because none of these children had symptoms that are associated with systemic yeast infection, such as fever. Although stool testing for yeast was not performed on this study group, results of stool yeast enumeration and putative yeast metabolites in urine have been compared in a substantial number of children with autism since this study; a general correspondence has been found in the 2 methods, indicating that the increased metabolites are likely to be derived from a GI yeast over growth. Both elevated arabinose and tartaric acid in the urine are associated with increased numbers of multiple species of Candida in stool samples.
The metabolites evaluated in this study are not specific for autism. Elevations of both the putative yeast and clostridia metabolites have been found to be common in the urine samples of many patients with many neuropsychiatric diseases, including pervasive developmental delay, child and adult psychosis, depression, Rett syndrome, seizure disorders, attention deficit hyperactivity, and Alzheimer’s disease. The findings in this study may open the investigation of the immune system and metabolites from the GI flora in these neuropsychiatric diseases as well. The putative yeast and clostridia metabolites were not elevated in urine samples of children with Down’s syndrome, but were elevated in urine samples of children with Down’s syndrome who also have autism.
The furan compounds are known fungal metabolites,18-20 but the origin of these compounds in urine is controversial.21-24 Speculation includes the possibility that they are artifacts derived from certain sugars, compounds formed from bakery products, and food precursors. Furan fatty acids have been detected in almost all living creatures including fish, crayfish, amphibians, reptiles, and mammals (including man), as well as in plants.23 The reduction of these compounds after nystatin therapy in this study seems consistent with a significant fungal origin for at least a portion of these compounds in urine. A large number of studies have indicated a wide number of abnormalities of the immune system in autism,25-29 including IgG deficiency, IgA deficiency, IgG subclass deficiency, myeloperoxidase deficiency (a genetic defect in an enzyme of the leukocytes that produces hypochlorite ions to kill yeast), and a deficiency in complement C4b. The 2 brothers with autism in which these metabolites were first reported1 both had abnormally low concentrations of serum IgG. Autism has also been diagnosed in other children with defined inborn errors of metabolism, such as biotinidase deficiency and isovaleric acidemia, in which yeast infections are common (J. Lombard, oral and written communication, 1997). Furthermore, the success of Gupta et al25 in treating the symptoms of autistic children with gamma globulin therapy indicates an immune abnormality in autism.
Based on these findings and the findings of abnormal organic acids in this study, we propose a model for autism
(Figure 3). According to this model, immune deficiencies, which may be genetic or acquired, lead to an increased frequency of infections that are almost always treated with broad-spectrum oral antibiotics. As a result of these antibiotics, a proliferation of yeasts and bacteria such as Clostridium occur in the GI tract; these organisms may eventually colonize the regional lymph nodes surrounding the GI tract. Furthermore, many isolates of Candida albicans produce gliotoxins30,31 and other immunotoxins32,33 that impair the immune system and increase the likelihood of additional infections, which lead to additional antibiotic usage and greater proliferation of yeasts and antibiotic resistant bacteria, setting up a vicious cycle. These organisms produce high amounts of abnormal carbohydrates, such as arabinose; Krebs cycle analogs, such as tartaric and citramalic acids; and compounds derived from phenylalanine (3-(3-hydroxyphenyl-3-hydroxypropionic acid) that are biochemically similar to the phenylalanine-and tyrosine-derived neurotransmitters. These compounds are absorbed into the bloodstream and may alter behavior; they may also produce neurotoxins in both the GI tract and in the regional lymph nodes that are absorbed into the circulation, also affecting behavior. If this model is correct, efforts to locate a single autism gene would fail since any genetic factor that severely impairs the immune system may eventually lead to the proliferation of antibiotic-resistant yeasts and bacteria, which then alter behavior through the excretion of their products. A portion of children with autism do not have a history of frequent infections. These children might have been exposed to yeast infection prenatally or developed abnormal microbial flora because of immune deficiencies rather than from repeated antibiotic therapy. This model is not inconsistent with other research findings in autism such as abnormal neuroanatomical findings, abnormal electroencephalogram results, and abnormal brain scans.
Similar abnormalities were found in PKU, even though the primary abnormality is a genetic defect in a single enzymatic reaction. There is no inherent reason that dramatic biochemical changes in multiple biochemical systems caused by microorganisms would not be expected to alter brain structure and function. In PKU, correction of the metabolic defect by restriction of phenylalanine during infancy allows for normal development; retardation occurs if dietary intervention occurs too late. If abnormally elevated metabolites cause autism, it is reasonable that elevations of these compounds would have maximum negative impact during periods of critical brain growth and development. As in PKU, metabolic intervention in autism might only be possible in the early stages of the disorder before the brain has matured. The differences in severity of disease and individual differences in symptoms might be due to different combinations of metabolites, how elevated they are, the duration of the elevation, the age at which the metabolites become abnormally elevated, and the susceptibility of the individual developing nervous system to the different microbial metabolites. Indeed, these differences may even determine which disease is manifested. Furthermore, the concentration of these microbial products is not trace amounts on the metabolic scale.
One nonstudy child with autism evaluated in our laboratory had a urine tartaric acid concentration (6000 mmol/mol creatinine) that was nearly 400 times the upper limit of normal after the use of multiple oral antibiotics. The value normalized after several weeks of antifungal therapy. Many of the concentrations of the microbial compounds reported here may exceed even the concentrations of the predominant mammalian organic acids in urine. Furthermore, many other metabolic products of microbial origin such as amino acids and lipids were not measured with the present organic acid test and should also be evaluated. It is likely that these abnormalities, some of which have been known for decades, have been ignored for so long by almost all of the researchers in the field of metabolic diseases because of the intense focus on finding new inborn errors of metabolism. By definition, abnormal microbial products are not due to a genetic defect in a human biochemical pathway. However, most researchers in the field of metabolic disorders also make the unwarranted assumption that microbial metabolites are metabolically and physiologically inert. Instead, the human and the microorganisms in the GI tract are an integrated biochemical system, the functions of which are interdependent.
The exact biochemical role of arabinose is unknown, but a closely related yeast alcohol arabitol has been used as a biochemical indicator of invasive Candidiasis.34-36 Elevated arabitol was not found in any of the urine samples tested in this study and arabinose was not found in the culture media of multiple isolates of Candida albicans isolated from stool samples of autistic children (W. Shaw, unpublished data, 1995). Arabitol produced by yeast in the intestinal tract may be absorbed into the portal circulation and then converted to arabinose by the liver. A nonstudy autistic child with the highest level of urine arabinose (over 40 times the upper limit of normal) had chronic hypoglycemia (blood glucose 20-50 mg/dL) for nearly 2 years. This child’s urine arabinose remained extremely elevated in repeated testing over the same period. The blood sugar of the child returned to normal for the first time in 2 years after 1 week of nystatin therapy concomitant with a marked decrease in urine arabinose, indicating a possible inhibition of gluconeogenesis by the arabinose. Similar hypoglycemia occurs in inborn errors of fructose metabolism, in which fructose inhibits gluconeogenesis,37 and it is possible that children with autism might be deficient in one or more enzymes involved in the metabolism of pentoses. Elevated protein-bound arabinose has been found in the serum glycoproteins of schizophrenics,38 and in children with conduct disorders39 and alteration of protein function by arabinose might be another mechanism by which arabinose might affect biochemical processes.
Arabinose, a sugar aldehyde or aldose, reacts with the epsilon amino group of lysine in a wide variety of proteins and may then form cross-links with arginine residues in an adjoining protein,40 thereby cross-linking the proteins and altering both biological structures and functions of a wide variety of proteins (Figure 4). This adduct of arabinose, lysine, and arginine is called a pentosidine (Figure 4). The epsilon amino group of lysine is a critical functional group of many enzymes to which pyridoxal (vitamin B6), biotin, and lipoic acid are covalently bonded during coenzymatic reactions41; the blockage of these active lysine sites by pentosidine formation may cause functional vitamin deficiencies even when nutritional intake is adequate. In addition, this epsilon amino group of lysine may also be important in the active catalytic site of many enzymes. Protein modification caused by pentosidine formation is associated with crosslink formation, decreased protein solubility, and increased protease resistance. The characteristic pathological structures, called neurofibrillary tangles associated with Alzheimer’s disease contain modifications typical of pentosidine formation. Specifically, antibodies against pentosidine react strongly to neurofibrillary tangles and senile plaques in brain tissue from patients with Alzheimer’s disease.42 In contrast, little or no reaction is observed in apparently healthy neurons of the same brain. Thus, it appears that the neurofibrillary tangles of Alzheimer’s disease may be caused by the pentosidines. The modification of protein structure and function caused by arabinose could account for the biochemical and insolubility properties of the lesions of Alzheimer’s disease through the formation of protein cross-links. Similar damage to the brains of autistic children might also be due to the pentosidines; neurofibrillary tangles have been reported in the brain tissue of an individual with autism.43 Since vitamin B6 reacts with the same critical epsilon amino group of lysine, the beneficial effects of vitamin B6 in autism reported in multiple studies44 may be mediated by prevention of further pentosidine formation. Analysis of brain tissue of people with autism for increased brain pentosidines could be invaluable in the confirmation of this hypothesis.
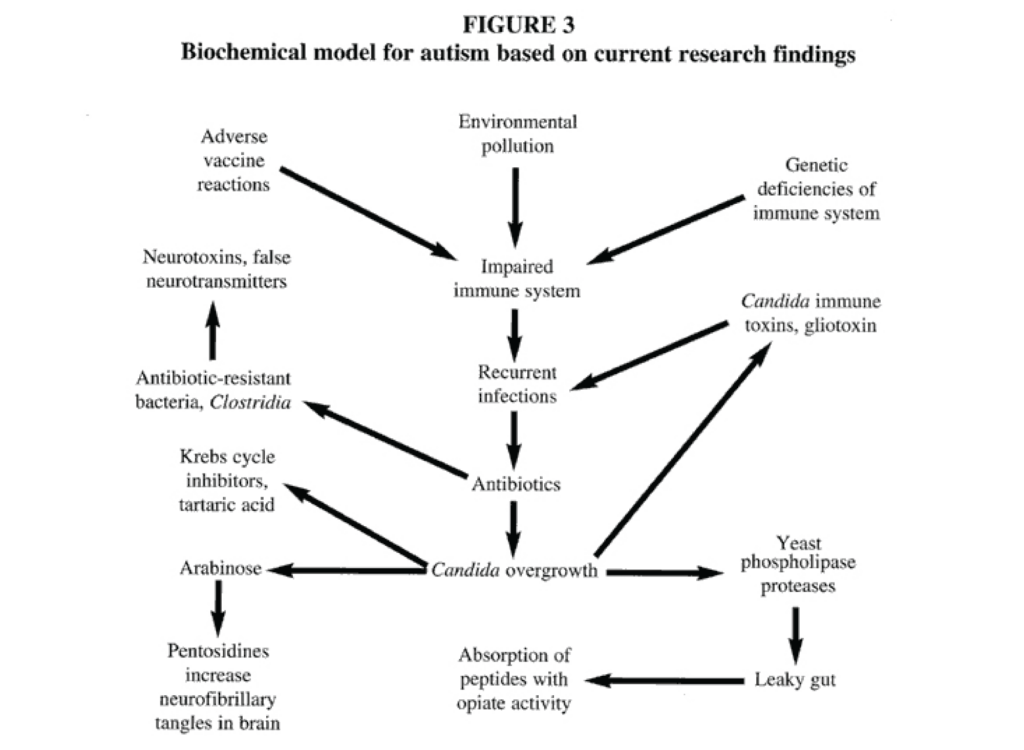
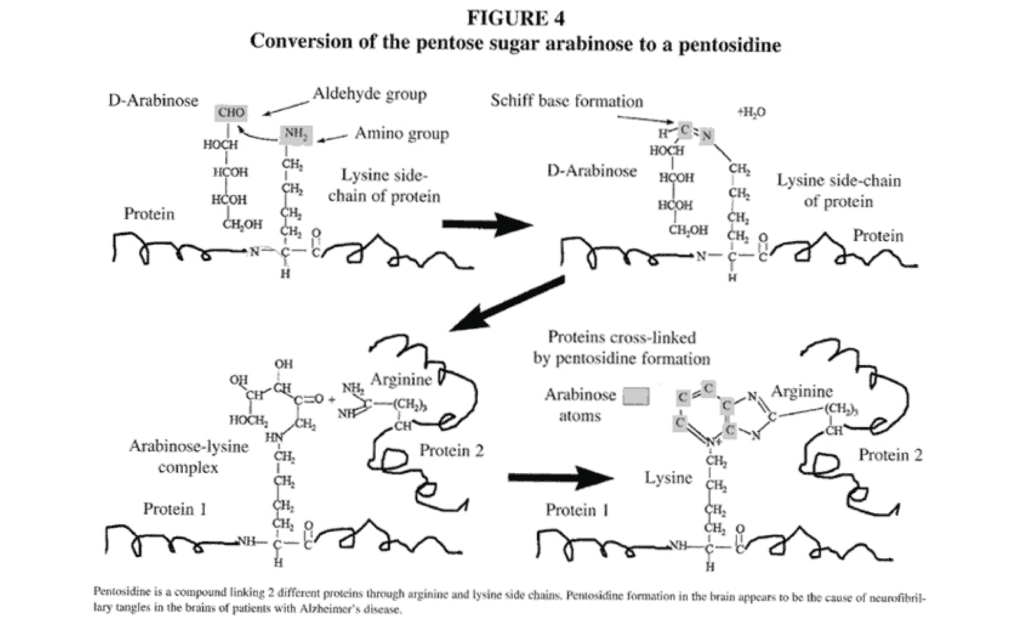
Horowitz et al45 found that women with vulvovaginitis due to Candida had elevated arabinose in the urine; restriction of dietary sugar brought about a dramatic reduction in the incidence and severity of the vulvovaginitis. Thus, one of the mechanisms of action of antifungal drug therapy for autism might be to reduce the concentration of an abnormal carbohydrate produced by the yeast that can not be tolerated by the child with defective pentose metabolism. Arabinose tolerance tests should be used to determine rapidly if such biochemical defects are present in children with autism.
Some of the other metabolites may also be due to a new inborn error of mammalian metabolism and/or a reduced ability to detoxify the microbial compounds. An analog of the Krebs cycle intermediate 2-oxoglutaric acid, 3-oxoglutaric acid, was first reported by Shaw et al1 as elevated in 2 brothers with autism, but was not previously known. This compound is not unique to autism, and in this study, it was found to be elevated in urine samples of several nonautistic children with seizure disorders, A child with seizures but not autism had the highest concentration of 3-oxoglutaric acid in a urine sample (40 mmol/mol creatinine), and had severe brain-demyelinating disease as judged by magnetic resonance imaging. This compound was elevated (concentration > 0.5 mmol/mol creatinine) in 5 of 23 (21.7%) of the children with autism in this study, and it was elevated in 25.6 % of children with autism (n=255) evaluated a laboratory for inborn errors of metabolism (W. Shaw, unpublished data, 1996), The highest value for a child with autism measured in this laboratory is a value of 30 mmol/mol creatinine. A closely related compound, 3-hydroxyglutaric acid, a metabolite of the amino acids tryptophan, lysine, and hydroxylysine, is elevated in the genetic disease glutaric acidemia type I,46 a neurological genetic disease due to a deficiency of glutaryl-CoA dehydrogenase, Abnormal elevations of the plasma l amino acids tryptophan and lysine have been reported in children with autism,47-50 The conversion of 3-hydroxyglutaryl CoA to 3-oxoglutaric would presumably be catalyzed by a 3-hydroxyacyl CoA dehydrogenase, one of the enzymes present on both a peroxisomal enzyme complex, as well as in the mitochondrion, which functions in the beta-oxidation of fatty acids.51 In other peroxisomal diseases, there is frequently a late onset of symptoms similar to many cases of autism, in which there is frequently developmental losses between 18 to 24 months of age. In the peroxisomal disorders, there is an accumulation of long-chain fatty acids. Autism is not the only developmental disorder associated with increased incidence and severity of otitis media, A number of studies have also indicated that increased incidence of attention deficit hyperactivity is associated with increased incidence of otitis media.52-56
Conclusions
Antifungal drug therapy may be a promising therapeutic method for the treatment of autism; however, a double-blind placebo study will be required to prove the effectiveness of this therapy unequivocally of glutaryl-CoA dehydrogenase, Abnormal elevations of the plasma l amino acids tryptophan and lysine have been reported in children with autism.47-50 The conversion of 3-hydroxyglutaryl CoA to 3-oxoglutaric would presumably be catalyzed by a 3-hydroxyacyl CoA dehydrogenase, one of the enzymes present on both a peroxisomal enzyme complex, as well as in the mitochondrion, which functions in the beta-oxidation of fatty acids.51 In other peroxisomal diseases, there is frequently a late onset of symptoms similar to many cases of autism, in which there is frequently developmental losses between 18 to 24 months of age. In the peroxisomal disorders, there is an accumulation of long-chain fatty acids. Autism is not the only developmental disorder associated with increased incidence and severity of otitis media, A number of studies have also indicated that increased incidence of attention deficit hyperactivity is associated with increased incidence of otitis media.52-56
Nystatin is not absorbed into the bloodstream at the doses employed in this study. If yeast or fungi are the source of abnormal metabolites, the significant reduction of some of these metabolites after nystatin therapy appears to indicate fungal overgrowth of the GI tract as the primary site of yeast or fungal colonization.
Organic acid testing appears to be a useful diagnostic test to indicate an overgrowth of yeast and bacteria in the GI tract of children with autism, to predict the response to antifungal drug therapy, and to indicate relapse following the cessation of antifungal drug therapy.
We are presently involved in evaluating the species of clostridia in stool samples of autistic children and in determining which species produce the 3-(3-hydroxyphenyl)-3-hydroxypropionic acid. Much additional research is needed to determine which species and/or subtypes of yeast or fungi and bacteria produce the compounds that are elevated in autism, to determine which compounds may be most important in the etiology of autism and how they are metabolized, to determine whether potential adverse effects caused by these compounds can be reversed by other therapies, to determine how antifungal drug and immunological therapy can be modified or combined to produce therapeutic results that have a greater therapeutic duration, and to determine if dietary measures can be used to potentiate the effects of antifungal drug therapy.
Acknowledgements
This research was supported by grants from Pfizer Pharmaceutical Company, Inc. and from the (Katherine B. Richardson Foundation. We thank Dr Michael Luxem for his assistance with the CARS evaluation.
References
- Shaw W, Kassen E, Chaves E. Increased excretion of analogs of Krebs cycle metabolites and arabinose in two brothers with autistic features, Clin Chem. 1995;41:1094-1104.
- Kontstantareas M, Homatidis S. Ear infections in autistic and normal children. J Autism Dev Dis. 1987;17:585-593.
- Kennedy M, Volz P. Dissemination of yeasts after gastrointestinal inoculation in antibiotic-treated mice. Sabouraudia. 1983;21:27-33.
- Danna P, Urban C, Bellin E, Rahal J. Role of Candida in pathogenesis of antibiotic associated diarrhoea in elderly patients. Lancet. 1991;337:511-514.
- Ostfeld E, Rubinstein E, Gazit E, Smetana Z. Effect of systemic antibiotics on the microbial flora of the external ear canal in hospitalized children. Pediatr. 1977;60:364-366.
- Kinsman OS, Pitblado K. Candida albicans gastrointestinal colonization and invasion in the mouse; effect of antibacterial dosing, antifungal therapy, and immunosuppression. Mycoses. 1989;32:664-674.
- Van der Waaij D. Colonization resistance of the digestive tract: mechanism and clinical consequences. Nahrung. 1987;31:507-517.
- Samonis G, Dassiou M. Antibiotics affecting gastrointestinal colonization of mice by yeasts. Chemotherapy. 1994;6:50-52.
- Samonis G, Gikas A, Toloudis P. Prospective evaluation of the impact of broad-spectrum antibiotics on the yeast flora of the human gut. Eur J Clin Microbiol Infect Dis. 1994;13:665-667.
- Samonis G, Gikas A, Anaissie E. Prospective evaluation of the impact of broad-spectrum antibiotics on gastrointestinal yeast colonization of humans. Antimicroh Agents Chemother. 1993;37;51-53.
- Gorbach S, Chang T, Goldin B. Successful treatment of relapsing Clostridium difficile colitis with Laclohacilius GG. Lancet. 1987;ii:1519.
- Bennett M, Bhala A. Poirier S, Ragni M, Will; S, Hale D. When do gut flora in the newborn produce 3-phenylpropionic acid? Implications for early diagnosis of medium-chain acyl-CoA dehydrogenase deficiency. Clin Chem. 1992;38:278-281.
- Berg R. Promotion of enteric bacteria from the gastrointestinal tracts of mice by oral treatment with penicillin clindamycin, or metronidazole. Infect Immun. 1981;33:854-861.
- Finegold S. Anaerobic infections and Clostridium difficile colitis emerging during antibacterial therapy. Scand J Infect Dis Suppl. 1986;49:160-164.
- Bartlett J. Antibiotic-associated diarrhea. Clin Infect Dis. 1992;15:573-581.
- American Psychiatric Association. Diagnostic and Statistical Manual of Mental Disorders DSM-1V. 4th ed. Washington DC; American Psychiatric Association; 1994.
- Schopler E, Reichler RJ, Rochen Renner B. CARS: The Childhood Autism Rating Scale. Los Angeles, Calif: Western Psychological Services; 1988.
- Sumiki Y. Fermentation products of mold fungi IV. Aspergillus glaucus. I. J Agr Chem Soc Jap. 1929;5:10. Abstract. 19. Sumuki Y. Fermentation products of molds. J Agr Chem Soc Jap. 1931;7: 819. Abstract.
- Kawarda A, Takahoshi N, Kitamura H, Seta Y, Takai M, Tamura S. Biochemical studies on bakanae fungus. Bull Agr Soc Jap. 1955; 19:84. Abstract.
- Mrochek J, Rainey W. Identification and biochemical significance of substituted furans in human urine, Clin Chem. 1972;18:821-828.
- Pettersen J, Jellum E. The identification and metabolic origin of 2-furoylglycine and 2,5-furandicarboxylic acid in human urine, Clin Chim Acta. 1972;41:199-207.
- Hanneman K, Puchta V, Simon E, Ziegler H, Ziegler G, Spiteller G. The common occurrence of furan fatty acids in plants. Lipids. 1989;24:296-298.
- Henderson S, Lindup E. Renal organic acid transport: uptake by rat kidney slices of a furan dicarboxylic acid which inhibits plasma protein binding of acidic ligands in uremia. J Pharmacol Exp Ther. 1992;263:54-60.
- Gupta S, Aggarwal, Heads C. Dysregulated immune system in children with autism: beneficial effects of intravenous immune globulin on autistic characteristics. J Autism Dev Dis. 1996;26:439-452.
- Warren RP, Foster A, Margaretten NC, Pace NC. Immune abnormalities in patients with autism. J Autism Dev Dis. 1986;16:189-197.
- Warren RP, Yonk J, Burger RA, Odell D, Warren WL. DR positive T cells in autism: association with decreased plasma levels of the complement C4B protein. Neuropsychobiology. 1995;39(2):53-57.
- Stubbs EG, Crawford ML, Burger DR. Vanderbark AA. Depressed lymphocyte responsiveness in autistic children. J Autism Child Schizophr. 1977; 7(1):49-55.
- Singh VK, Fudenberg HH, Emerson D, Coleman M. Immunodiagnosis and immunotherapy in autistic children. Ann NY Acad Sci. 1988;540:602-604.
- Shah D, Larsen B. Identity of a Candida albicans toxin and its production in vaginal secretions. Med Sci Res. 1992;20:353-355.
- Shah D, Larsen B. Clinical isolates of yeast produce a gliotoxin-like substance. Mycopathologia. 1991 ;116:203-208.
- Fischer A, Ballet J, Griscelli C. Specific inhibition of in vitro Candida-induced lymphocyte proliferation by polysaccharide antigens present in the serum of patients with chronic mucocutaneous candidiasis. J Clin Invest. 1978;62:1005-1013.
- Iwata K, Ichita K. Cellular immunity in experimental fungal infections in mice. Mykosen Suppl. 1978;1:72-81.
- Roboz J, Katz R. Diagnosis of disseminated candidiasis based on serum D/L arabinitol ratios using negative chemical ionization mass spectrometry. J Chromatogr. 1992;575:281-286.
- Wong B, Brauer K, Clemens J, Beggs S. Effects of gastrointestinal Candidiasis, antibiotics, dietary arabinitol, and cortisone acetate on levels of the Candida metabolite D-arabinitol in rat serum and urine. Infect Immunol. 1990;58:283-288.
- Larsson L. Determination of microbial chemical markers by gas chromatography-mass spectrometry-potential for diagnosis and studies on metabolism in situ. APMIS. 1994;102:161-169.
- Gitzelman R, Steinmann B, Van der Berghe G. Disorders of fructose metabolism. In: Scriver C, ed. The Metabolic Basis of Inherited Disease. 6th ed. New York, NY: McGraw Hill; 1989;399-424.
- Varma R, Hoshino A. Serum glycoproteins in schizophrenia. Carbohydr Res. 1980;82:343-351.
- Varma R, Michos G, Gordon B, Varma RS, Shirey R. Serum glycoproteins in children with schizophrenia and conduct and adjustment disorders. Biochem Med. 1983;30:206-214.
- Sell D, Monnier V. Structure elucidation of a senescence cross-link from human extracellular matrix; implication of pentoses in the aging process. J Biol Chem. 1989;264:21597-21602.
- Mahler H, Cordes E. Biological Chemistry. New York, NY: Harper and Row; 1966;525-553.
- Smith MA, Taneda S, Richey PL, et al. Advanced Maillard reaction end products are associated with Alzheimer disease pathology. Proc Nati Acad Sci USA. 1994;91:5710-5714.
- Hof PR, Knabe R, Bovier P, Bouras C. Neuropathological observations in a case of autism presenting with self-injury behavior. Acta Neuropathol (Berl). 1991;82: 321-326.
- Rimland B. New hope for safe and effective treatments for autism. Autism Res Rev Int. I994;8:3.
- Horowitz B, Edelstein S, Lipman L. Sugar chromatography studies in recurrent vulvovaginitis. J Reprod Med. 1984;29:441 -443.
- Stokke 0, Goodman S, Thompson J, Miles B. Glularic aciduria: presence of glutaconic and beta-hydroxyglutaric acids in urine. Biochem Med. 1975; 12:386-391.
- Zavala M, Ortega P, Montiel N, et al. Plasma amino acid levels in normal children and children with autism. In: Autism Society of America. 1996 Autism Society National Conference Proceedings. Bethesda, Md: Autism Society of America; 1996:243-246.
- Balasz A. Current concept of autism. Orv Hetil. 1991;132:2827-2835.
- Moreno H. Clinical heterogeneity of the autistic syndrome: a study of 60 families. Invest Clin. 1991;33(1):13-31.
- Kotsopoulos S, Kutty K. Histidinemia and infantile autism. J Autism Dev Dis. 1979;9(1):55-60.
- Watkins P, McGuiness M, Raymond G, et al. Distinction between peroxisomal bifunctional enzyme acyl-CoA oxidase deficiencies. Ann Neurol. 1995; 38:473-477.
- Roberts J, Burchinal M, Campbell F. Otitis media in early childhood and patterns of intellectual development and later academic performance. J Pediatr Psychol. 1994;19:347-367.
- Hagerman R, Falkenstein A. An association between recurrent otitis media in infancy and later hyperactivity. Clin Pediatr. 1987;26:253-257.
- Teele D, Klein J, Rosner B, The Greater Boston Study Group. Otitis media with effusion during the first years of life and development of speech and language. Pediatrics. 1984;74:282-287.
- Silva P, Chalmers D, Stewart I. Some audiological, psychological, educational, and behavioral characteristics of children with bilateral otitis media with effusion: a longitudinal study. J Learn Disabil. 1986;19:165-169.
- Sak R, Ruben R. Effects of recurrent middle ear effusion in preschool years on language and learning. Dev Behav Pediatr. 1982;3(1):7-11.