William Shaw, PhD; Matthew Pratt-Hyatt PhD
Townsend Letter – November 2019
Abstract
Seven different mycotoxins, including aflatoxin M1, ochratoxin A, sterigmatocystin, zearalenone, roridin E, verrucarin A, and enniatin B1 that have been associated with human illness were tested by LC/MS/ MS in urine samples of a control group of 89 healthy adult volunteers and 103 adult patients suspected of mold illness based on clinical symptoms assessed by their physicians and findings of mold growth in their homes or offices. In addition, markers of gastrointestinal mold and yeast colonization, mitochondrial function, and glutathione status markers were assessed in the same urine samples by GC/MS. Values for all the mycotoxins tested were significantly increased in the mold patient group compared to the control group. Markers for mold colonization of the gastrointestinal tract were all significantly more elevated in the mold-exposed group compared to the control group. Lactic acid, oxalic acid, and pyroglutamic acid were all significantly higher in the mold- exposed patient group compared to the control group. Succinic acid, a marker of mitochondrial function was also higher in the mold- exposed group but did not reach statistical significance. Elevated markers of Fusarium and Aspergillus gastrointestinal colonization in mold- exposed patients indicates a need for antifungal treatment for successful treatment of mold-exposed patients.
Introduction
Mycotoxins are physiologically active metabolites released by specific strains of filamentous fungi. Over 400 different mold metabolites have been identified, however to date only several dozen have been shown to have significant toxic potency in humans.1 Routes of exposure include inhalation, absorption through the skin, exposure to mold from water-damaged buildings, occupational exposure, ingestion of mold-contaminated food,2,3 and mycotoxins produced by mold in the gastrointestinal tract or other organs. The mycotoxins that we evaluated in this study, including aflatoxin M1 (AFM1), ochratoxin A (OTA), sterigmatocystin (STC), zearalenone (ZEA), roridin E (ROE), verrucarin A (VER), and enniatin B (ENB), have multiple toxic properties which elicit health effects in patients. These properties include cytotoxicity, carcinogenicity, teratogenicity, hepatotoxicity, nephrotoxicity, neurotoxicity and immuno- suppression.4-6
Exposure to mycotoxins can lead to acute as well as chronic toxic effects, which are dependent on the mycotoxin type, the amount, length of the exposure, and the health, age, and sex of the patient. Dietary deficiency, co-existing health conditions, and environmental/chemical exposure potentially create a compounding effect which can impact the severity of symptoms. Mycotoxins have been found in multiple different tissues with the highest concentrations found in liver and kidneys.7 Other tissues that can contain significant amounts of mycotoxins include brain, muscle, fat, skin, myocardium, gastric mucosa, and bone marrow.7 Mycotoxin exposure can lead to many different types of acute or chronic diseases.8,9 Symptoms of mycotoxin exposure can include cough, irritation of the eyes and skin, fatigue, migraines/headaches, joint pain, and neurological illnesses such as Alzheimer’s disease, Parkinson’s disease, and multiple sclerosis.10-14
Monitoring mycotoxins and their metabolites in human samples to assess the exposure of individuals to mold is one of the first steps in determining the proper treatment for these patients. Recent advances in LC-MS/MS techniques now allow urinary biomarker assessments at environmentally relevant concentrations.10,15-17 Studies have shown that mycotoxins are produced by multiple species of mold, including those from Stachybotrys, Aspergillus, Fusarium, and Penicillium9 genera. Some of the important mycotoxins produced by these molds include aflatoxin M1 (AFM1) and ochratoxin A (OTA) from Aspergillus and Penicillium, zearalenone (ZEA) and enniatin B (ENB) from Fusarium, sterigmatocystin (STC) from Penicillium, roridin E (ROE) and verrucarin A (VER) from Stachybotrys).15 Urine has been determined to be the preferred matrix for mycotoxin analysis because most mycotoxins are metabolized and excreted via urine.18
In addition to monitoring these toxins in the urine of exposed and control patients, we examined metabolic markers to assess their relevance to alterations of other biochemical functions, which could assist in determining proper treatment for mold exposure. GC/MS instrumentation was used to measure known small molecule biomarkers and to assess their correlation with mold and mycotoxin exposure. The specific urine markers that were analyzed have been shown to give insight on mold colonization of the gastrointestinal tract, yeast coinfection, mitochondrial damage, and glutathione usage.
Studies have shown that multiple species of mold can colonize different tissues in the body including the lungs, gastrointestinal tract, and sinus cavities and a recent study found Aspergillus and Penicillium species to be present in the gastrointestinal tracts of healthy volunteers.19 Some of the most frequently diagnosed fungal pathogens come from the genera Aspergillus and Candida. To test for colonization of Aspergillus, we measured 5-hydroxymethyl-2-furoic and furan-2,5-dicarboxylic acids.20-21 We measured tricarballylic to indicate the presence ofFusarium species.22
Because of the immunosuppressive abilities of mycotoxins released from Aspergillus and other colonizing mold species, Candida can frequently coinfect a patient.23 Species of Candida are frequently opportunistic pathogens that may cause various infections from oral candidiasis to systemic blood stream infections.24 There are over 200 species of invasive Candida. Candida albicans is the most prevalent fungal pathogen of humans, however Candida glabrata, Candida krusei, Candida tropicalis, and Candida parapsilosis can also cause human candidiasis.25 Candida can colonize skin, mucosal surfaces, and the gastrointestinal tract. As a marker for Candida colonization, we measured citramalic acid, which has been shown to be produced by various species of yeast.26
Mitochondria are double membrane- bound organelles that generate the cell’s energy through the production of adenosine triphosphate (ATP). The mitochondria produces other forms of energy through the citric acid cycle (TCA) in the form of NADH and FADH.27 Previous studies have shown a role for mitochondria in mycotoxin toxicity.28-32 OTA exposure can lead to mitochondrial transmembrane potential loss and caspase-3 activation. Multiple cellular changes occur following OTA exposure, which include decrease of Bcl-xL, survivin, (which lead to increases of apoptosis) and IL-2 and an increase of tumor necrosis factor alpha (TNF-α).30,32 Mycotoxinsalsoinhibitmitochondrial efficiency by inhibiting mitochondrial translation. This repression of mitochondrial translation leads to increase in mitochondrial oxidative stress and contributes to mycotoxin sensitivity.29 To determine if patients exposed to mycotoxins are exhibiting mitochondrial stress, we decided to measure the organic acids lactic acid and succinic acid, which have been shown to be elevated in patients exhibiting mitochondrial disease.33-34
Glutathione is a ubiquitous tripeptide that is present in almost all cells in high concentrations. Reduced glutathione is produced through two mechanisms in the body, de novo synthesis and recycling of oxidized glutathione dimers, GSSG. Its main function is to protect cells from toxic effects of oxygen-derived free radicals. There are many glutathione-dependent enzymes that perform a host of different regulatory and detoxification processes throughout the body.35 One of these is a family of enzymes called glutathione transferases (GST). These enzymes utilize glutathione to conjugate and detoxify drugs and other xenobiotics. This pathway is one of the main pathways for the detoxification of mycotoxins. Depletion of glutathione or inhibition on the transferase enzymes can cause patients to become more susceptible to mycotoxin induced injury.36-37 Patients that have higher utilization of glutathione have higher elimination of pyroglutamic acid in their urine.38 Measurement of pyroglutamic acid can give us insight into the patient’s utilization of glutathione.
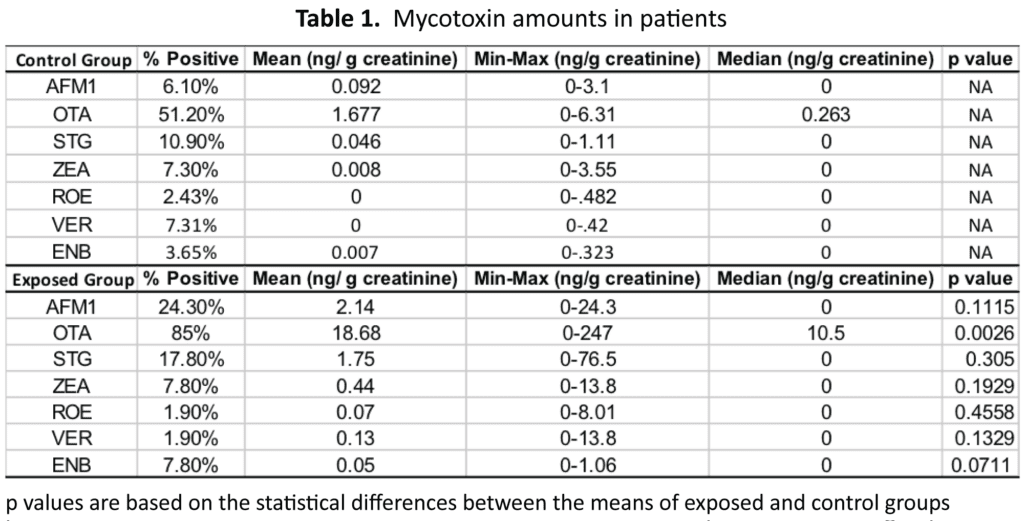
Results of Mycotoxin Analysis
AFM1, OTA, STC, ZEN, ROE, VER, and ENB were analyzed by LC-MS/MS in urine for both the control group as well as the mycotoxin exposed group. For this purpose, a previously published method was used.39 In Tables 1 and Figure 1, we detail the results from the control group and the mycotoxin exposed groups respectively. A positive value was any detectable value above out limit of quantification, which was 1.3 ng/g creatinine for AFM1, 1.2 ng/g creatinine for OCA, 0.1 ng/g creatinine for STC, 0.5 ng/g creatinine for ZEA, 0.75 ng/g creatininine for VRA, and 0.009 ng/g creatinine for ENB. For AFM1, the mycotoxin was detected almost four times more often in the exposed group vs the control (24.3% vs 6.1%). The mean value for the exposed group was 23 times greater (2.14 vs 0.092 ng/g creatinine) than the control group. For OTA, the exposed group had more patients with detectable OTA than the control group. The mean for OTA was 11 times higher in the exposed group vs the control (18.68 vs 1.677 ng/g creatinine). STG was positive more often in the exposed group than the control group (17.8 vs 10.9%), and the mean value was 38 times higher in the exposed group (1.75 vs 0.046 ng/g creatinine).
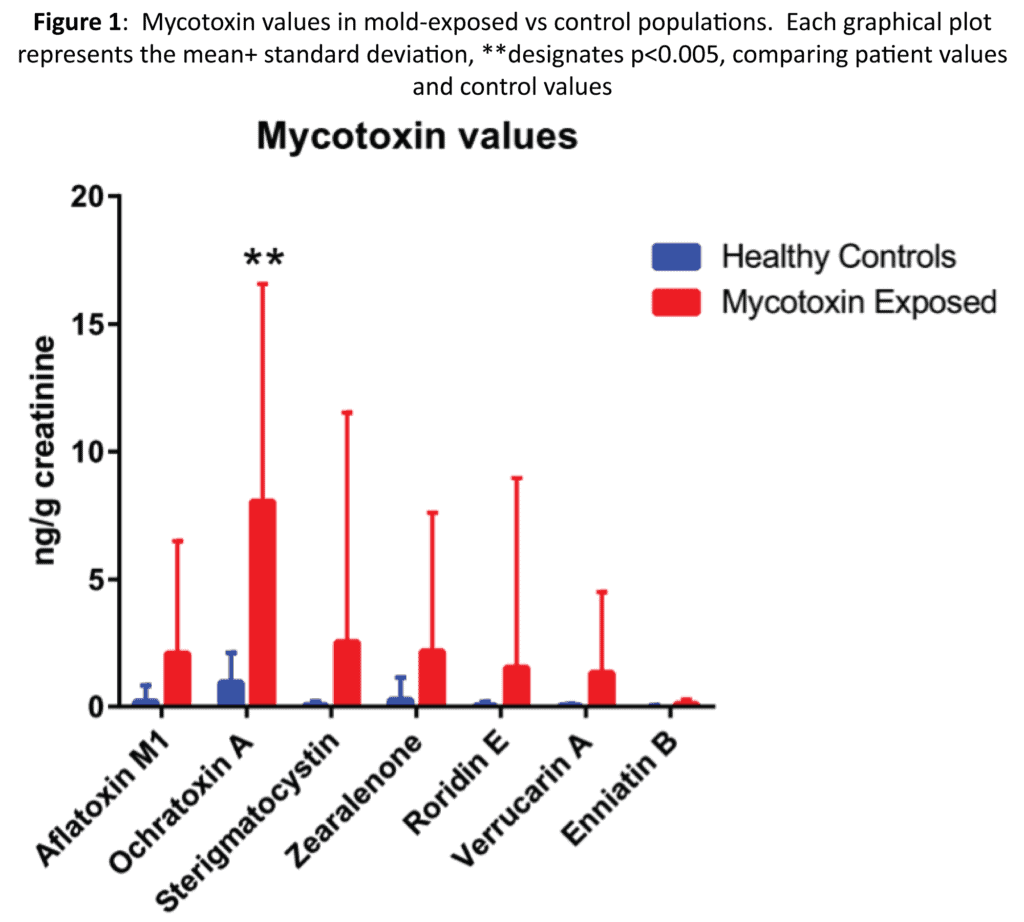
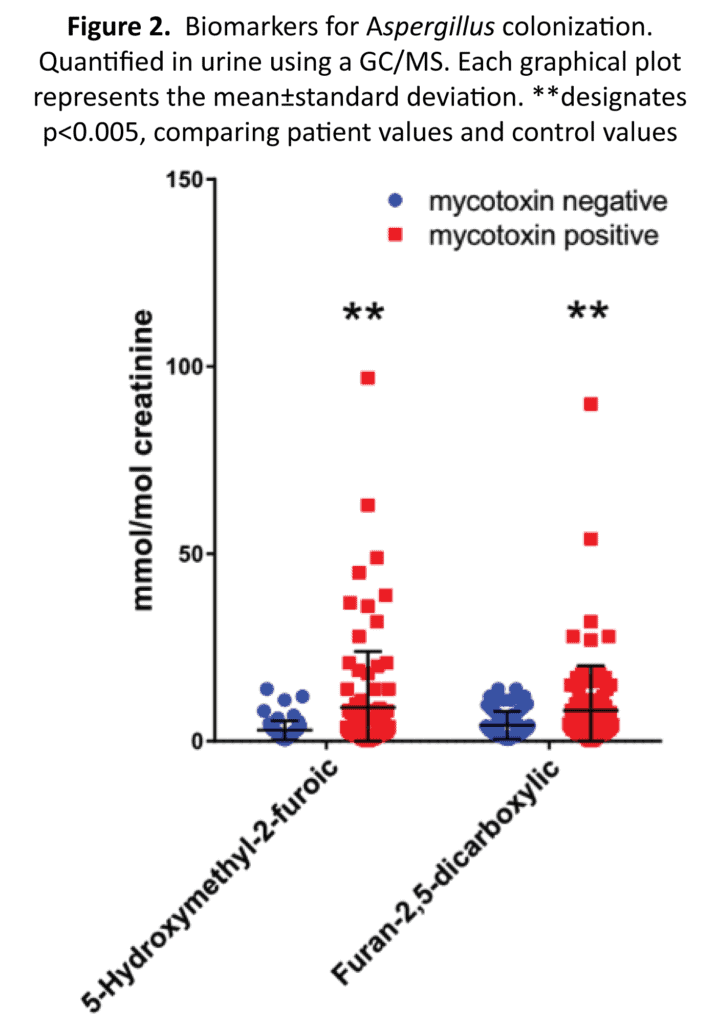
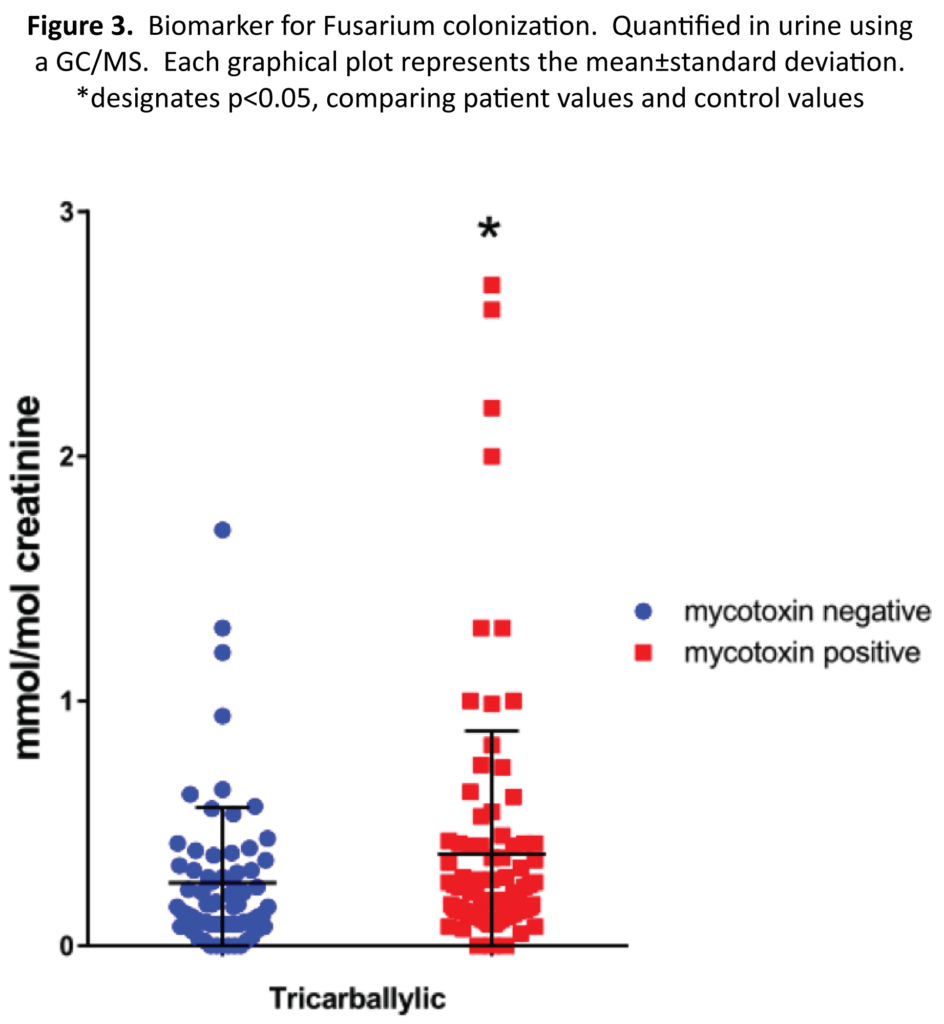
Biochemical Markers Associated with Mycotoxin Exposure
Mycotoxin exposure can affect many different systems in the body. Measurements of these effects on patients can help in treatment. Utilizing GC/MS, we measured other markers that could be altered by exposure to mycotoxins. The markers that we were most interested in testing were related to Aspergillus colonization, yeast colonization, mitochondrial status, and glutathione status.
To determine if markers for Aspergillus or Fusarium growth is more abundant in patients exposed to mycotoxins vs controls, we decided to measure the amounts of three different markers. Two metabolites, 5-hydroxymethyl-2-furoic and furan- 2,5-dicarboxylic acids, were analyzed as markers for Aspergillus (Figure 2) and tricarballylic measurements were used as an indicator for Fusarium (Figure 3). The 5-hydroxymethyl-2-furoic acid values were higher in patients exposed to mold vs the control patients (9.11 vs 2.91 mmol/mol of creatinine) (p value= 0.00075). Furan 2,5-dicarboxylic acid was also found to be higher in patients exposed to mold. In the mold-exposed patients, furan-2,5-dicarboxylic acid was 8.23 and in the control group it was 4.32 ng/g creatinine (p value = 0.00904). The tricarballylic acid mean was found to be twice as much in the mold-exposed group versus the control group (0.46 vs 0.22 mmol/mol of creatinine) (p value = 0.0493). One additional marker that we measured was oxalic acid. Oxalic acid is a biproduct from Aspergillus.40 Oxalic acid in the colonized group was significantly higher than the control group (Figure 4) (137.7 vs 72.6 mmol/mol of creatinine) (p value=0.0029).
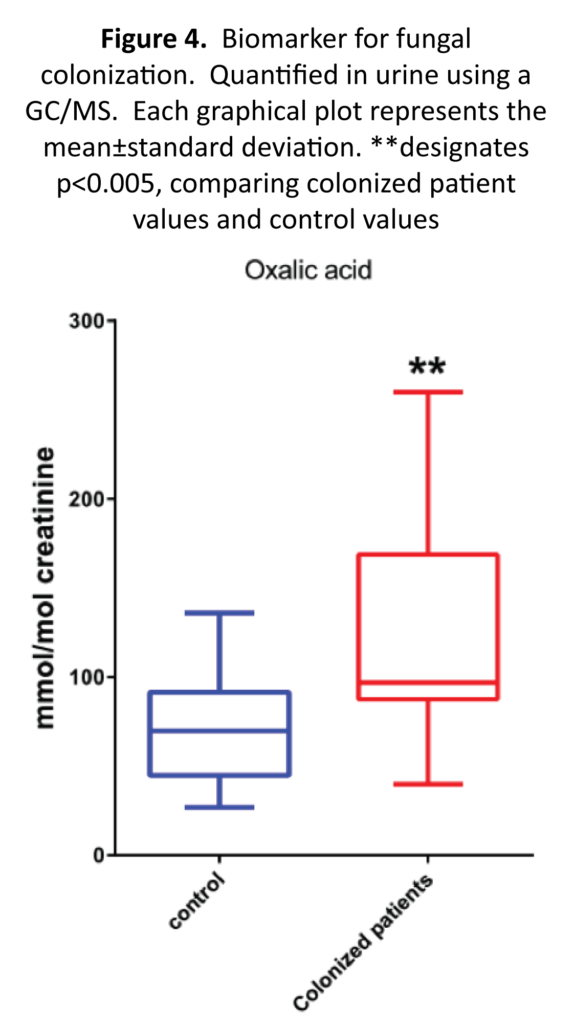
Candida as well as other pathogens can infect a patient that has been exposed to mycotoxins.23 One reason for this increased risk of infection is that many mycotoxins possess immunosuppressive affects.41 To measure the amount of Candida residing in a patient, we selected citramalic as a marker, which has been shown to be produced by multiple strains of yeast (Figure 5).26 Citramalic was significantly higher in the mold-exposed group versus the control group (1.45 vs 1.02 mmol/mol of creatinine) (p value = 0.00191).
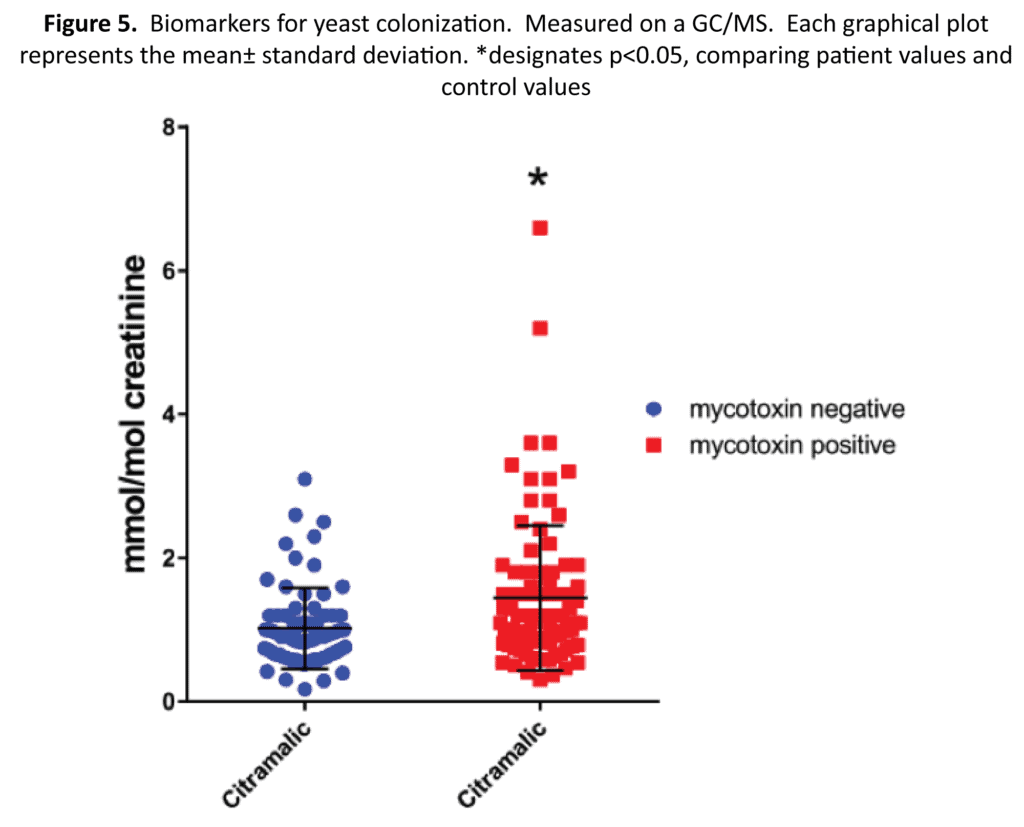
Fatigue is a common symptom of mold exposure, which can be attributed to decreased mitochondrial efficiency caused by mycotoxins.14 To test for mitochondrial stress, lactic acid and succinic acid were measured (Figure 6). Lactic acid is produced from pyruvate by lactate dehydrogenase when additional energy production is needed. Lactic acid is then either converted back to pyruvate or converted to glucose through gluconeogenesis. Succinic acid is generated in mitochondria through the tricarboxylic acid cycle. Succinate is then converted to fumarate by succinate dehydrogenase. In patients exposed to mycotoxins, lactic acid was significantly higher in their urine than the control group (29.8 vs 18.44 mmol/mol of creatinine) (p value = 0.00229). For succinate, the observed value for the exposed group was higher than the control (9.56 vs 6.35 mmol/mol of creatinine); however, the value was not statistically significant (p value 0.12).
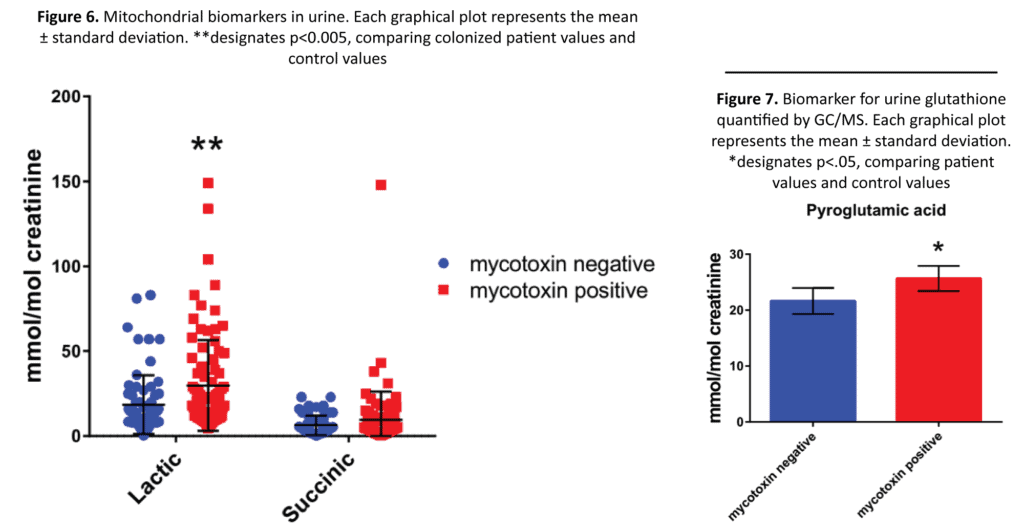
Glutathione conjugation is one of the primary pathways for the detoxification of mycotoxins. Mycotoxin exposure can lead to the depletion of glutathione stores, which will limit the body’s ability to detoxify multiple different xenobiotics. Depletion of glutathione or inhibition on the transferase enzymes can lead patients to become more susceptible to mycotoxin induced injury.36,37 Measuring pyroglutamic acid (5-oxoproline) in patient urine may give us insight into which patients are depleted of glutathione and are more at risk for mycotoxin inducted injury. When glutathione concentration is reduced, γ-glutatmylcysteine formation increases and this dipeptide is converted to pyroglutamic acid in the plasma; some of the pyroglutamic acid is excreted in urine.42 Patients exposed to mold had significantly higher amounts of pyroglutamic acid in their urine compared to controls (Figure 7) (25.69 vs 21.63 mmol/mol of creatinine, p value = 0.01461)
Conclusions
Mold and mycotoxin exposure can affect multiple systems, including detoxification, immune function, and neuronal function, among others. Mycotoxins attack multiple cell types through their interactions with mitochondria, nuclear transcription factors, as well as DNA.27,30,32,37 We employed LC/MS/MS to quantitate mycotoxin exposure and GC/MS to measure other biomarkers that may indicate severity of effects on patients from the mycotoxins.
Ochratoxin was the most prevalent mycotoxin found in mold-exposed patients (Table 1). The mold-exposed group had detectable amounts of ochratoxin in 85% of the patients; however, the control group also had detectable amounts in over half of that population (51.2%). The mold-exposed group had over 10-fold increase in detectable ochratoxin compared to the control group (18.68 vs 1.68 ng/g creatinine). Other mycotoxins that were increased in the mold-exposed group were aflatoxin M1 and sterigmatocystin.
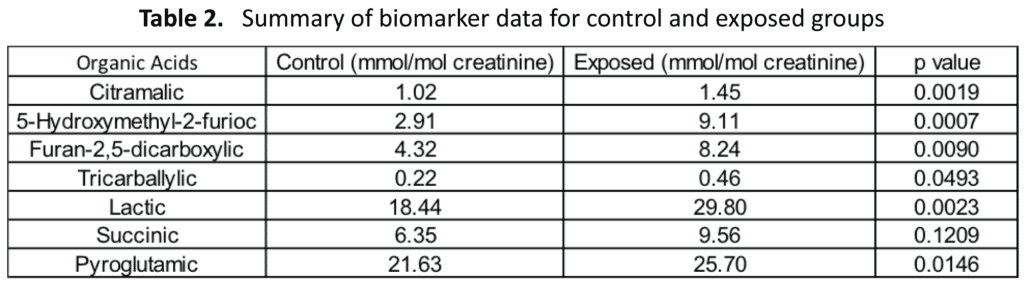
For biomarker analysis by GC/MS, there were several significant differences between the exposed group versus the control group (Table 2). The Aspergillus and Fusarium markers 5-hydroxymethyl-2-furioc, furan-2,5-dicarboxylic, and tricarballylic acids were all significantly elevated in patients with mold exposure. A previous study indicated that one site of mold colonization was the gastrointestinal tract that was ascertained by treatment with the antifungal drug nystatin, which is not absorbed from the gastrointestinal tract. The fact that the mold markers in that study were significantly reduced (56-63%) after 10 days of nystatin indicated that at least a substantial portion of the molds producing these markers was from the gastrointestinal tract.43 The fact that the gastrointestinal tract was colonized with mold species also indicates that patients exposed to mold that has colonized the gastrointestinal tract might continue to have severe symptoms long after moving out of a mold-contaminated environment unless treated with antifungal agents to kill gastrointestinal molds.
Patients that were exposed to mold also had a higher instance of yeast colonization, which was measured by increased levels of the yeast metabolite citramalic. The mitochondrial marker lactic acid was significantly elevated in patients exposed to mold. Oxalic acid, a toxic metabolite of Aspergillus, was elevated in the patients who were colonized. Oxalic acid has been shown to have toxic effects on virtually every organ of the human body including urinary tract, cardiovascular system, brain, muscles, thyroid gland, and eyes.44 Lastly, pyroglutamic, a glutathione usage deficiency marker, was elevated in the patients exposed to mold versus our control samples. This was expected because glutathione is depleted in the detoxification of many mycotoxins.
This study demonstrates that patients that are exposed to mold have elevated mycotoxins in their bodies, which can be measured by urine excretion. These mycotoxins cause multiple effects in the body. We were able to measure some of these effects through different urinary biomarkers by GC/MS.
Materials and Methods
Selection of human subjects for test. This study enrolled a grand total of 185 adults, split into the self-reported mold-exposed group (n=103) and a healthy control group of adults at least 18 years old with no known mold exposure (n=82). Mold-exposed patients were reported by their medical practitioner to have symptoms that matched mold exposure.
Chemicals and reagents. Urinary organic acid standards were purchased from Sigma-Aldrich (St. Louis, MO, USA) and mycotoxin standards solutions of ZEA, OTA, STG, VER, and ROE were purchased from Romer Labs Diagnostic (Tulln, Austria). Standard solution of AFM1 was purchased from Sigma- Aldrich. Acetonitrile (ACN), ethyl acetate (EtOAc), methanol (MeOH), and chloroform (CHCl3) were purchased from Thermo-Fisher Scientific (USA). Solid standards were dissolved and combined into a multi-standard working solution for preparation of calibrants. Ultrapure water was produced by a Milli-Q system (Millipore, Bedford, MA, USA).
Equipment and conditions. Urinary organic acid analyses were performed on a gas chromatography-mass spectrometry (GC-MS) system. The GC-MS used was from Agilent (Palo Alto, CA, USA) and consisted of a 5977B mass spectrometer detector and a 7890B gas chromatograph. For GC-MS analysis, 1 μl of sample was injected onto a 10-m DB-1MS capillary column with a 0.10- mm diameter, with helium as the carrier gas. All experiments were done using electron-impact ionization with electron energy of 70 eV. The temperature program was started at 90oC, held for one minute after injection, and then increased to 280oC at a rate of 20oC/ min.
A Tokyo Boeki Chemistry Analyzer was used to determine creatinine (mg/ dL) using a modified Jaffe method with Pointe Scientific creatinine reagent. All results were normalized to creatinine to correct for hydration variation among participants.
Participants and urine collection. All the volunteers were informed on how to collect first morning urine samples. For analyses, 25 ml of urine was collected from each sample. The two groups of patients were healthy volunteers (82) and patients suspected to have mycotoxin exposure (103). Sample kits were mailed to participants’ homes and then overnighted to the lab. The detection and quantification of urinary mycotoxin metabolites was performed using a liquid chromatography tandem mass spectrometry (LC-MS/MS) system including binary pumps, auto injector, separation column, and MS/ MS detector LC-MS/MS analyses were performed on a triple quadrupole 8060 (Shimadzu, Kyoto, Japan). The method of analysis was detailed in a previous paper.39
Organic acid analysis. Urine organic acid analysis has historically been performed using GC/MS instrumentation. Organic acid analytes are extracted from urine and derivatized before being analyzed by GC/MS. This analysis was performed similarly as first described by Tanaka et al.45 Urine samples were prepared for GC/MS analysis using liquid-liquid extraction technique. Samples were stored under refrigeration (-20oC) until testing. A 1 mL aliquot of each urine sample was first incubated with 200 uL ethyl hydroxylamine hydrochloride (75g/L) at 60oC for 30 minutes to convert keto-acids to their ethoxime derivatives. The samples were then acidified to pH 1 with 100 uL 6N HCl to convert organic acids to an uncharged state before being extracted with ethyl acetate and ether. Solvent extracts were evaporated to dryness under nitrogen and reacted with BSTFA (1%TMCS) at 80oC for 10 minutes. Trimethylsilyl (TMS) derivatized samples were analyzed by GCMS with spectral confirmation against a library database. Quantitation was based on analyte specific calibrations using standards prepared at known concentrations. Low and high controls were prepared using pooled urine and standard-spiked urine, respectively.
Mycotoxin analysis. Samples were prepared as seen in De Santes et al.39
Statistical analyses. For the GC/MS and LC/MS/MS biomarker tests, t test with Bonferroni multiple comparisons test was performed to compare the results between the mold-exposed group and the control group. All analyses were performed with Prism version 6.07 software (GraphPad Software, La Jolla, CA, USA). A value of p< 0.05 was considered statistically significant.
References
- Smoragiewicz W, et al. Trichothecene mycotoxins in the dust of ventilation systems in office buildings. Int Arch Occup Environ Health. 1993, 65 (2), 113-7.
- Shoemaker RC, House DE. Sick building syndrome (SBS) and exposure to water-damaged buildings: time series study, clinical trial and mechanisms. Neurotoxicol Teratol. 2006, 28 (5), 573-88.
- Bennett JW, Klich M. Mycotoxins. Clin Microbiol Rev 2003, 16 (3), 497-516.
- Smith JE, et al. Cytotoxic fungal spores in the indoor atmosphere of the damp domestic environment. FEMS Microbiol Lett. 1992, 100 (1-3), 337-43.
- Richard JL. Some major mycotoxins and their mycotoxicoses- -an overview. Int J Food Microbiol. 2007, 119 (1-2), 3-10.
- Hsieh DP.Biological reactive intermediates of bisfuranoid mycotoxins. J Toxicol Sci. 1990, 15 Suppl 4, 152-8.
- Breitholtz-Emanuelsson A. et al. Synthesis of 14C-ochratoxin A and 14C-ochratoxin B and a comparative study of their distribution in rats using whole body autoradiography. Pharmacol Toxicol. 1992, 70 (4), 255-61.
- Hendry KM, Cole EC. A review of mycotoxins in indoor air. J Toxicol Environ Health 1993, 38 (2), 183-98.
- Tuomi T, et al. Mycotoxins in crude building materials from water-damaged buildings. Appl Environ Microbiol 2000, 66 (5), 1899-904.
- Martins IJ. Overnutrition Determines LPS Regulation of Mycotoxin Induced Neurotoxicity in Neurodegenerative Diseases. Int J Mol Sci 2015, 16 (12), 29554-73.
- Bredesen DE. Inhalational Alzheimer’s disease: an unrecognized – and treatable – epidemic. Aging (Albany NY) 2016, 8 (2), 304-13.
- Rautiala S, et al. Exposure to airborne microbes during the repair of moldy buildings. Am Ind Hyg Assoc J 1996, 57 (3), 279-84.
- Hodgson MJ, et al. Building-associated pulmonary disease from exposure to Stachybotrys chartarum and Aspergillus versicolor. J Occup Environ Med 1998, 40 (3), 241-9.
- Auger PL, Gourdeau P, Miller JD. Clinical experience with patients suffering from a chronic fatigue-like syndrome and repeated upper respiratory infections in relation to airborne molds. Am J Ind Med 1994, 25 (1), 41-2.
- Gerding J, Cramer B, Humpf HU. Determination of mycotoxin exposure in Germany using an LC-MS/MS multibiomarker approach. Mol Nutr Food Res 2014, 58 (12), 2358-68.
- Klapec T, et al. Urinary ochratoxin A and ochratoxin alpha in pregnant women. Food Chem Toxicol 2012, 50 (12), 4487-92.
- Ediage EN, et al. A direct assessment of mycotoxin biomarkers in human urine samples by liquid chromatography tandem mass spectrometry. Anal Chim Acta 2012, 741, 58-69.
- Warth B, et al. New insights into the human metabolism of the Fusarium mycotoxins deoxynivalenol and zearalenone. Toxicol Lett 2013, 220 (1), 88-94.
- Auchtung,TA, et al. Investigating Colonization of the Healthy Adult Gastrointestinal Tract by Fungi. mSphere 2018, 3 (2).
- Kimura Y, et al. Nematicidal activity of 5-hydroxymethyl-2- furoic acid against plant-parasitic nematodes. Z Naturforsch C. 2007, 62 (3-4), 234-8.
- Kaji A, et al. Four new metabolites of Aspergillus terreus. Chem Pharm Bull (Tokyo) 1994, 42 (8), 1682-4.
- Lia Y, et al. Tricarballylic ester formation during biosynthesis of fumonisin mycotoxins in Fusarium verticillioides. Mycology. 2013, 4 (4), 179-186.
- Richardson MD. Changing patterns and trends in systemic fungal infections. J Antimicrob Chemother. 2005, 56 Suppl 1, i5-i11.
- Marinho SA, et al. Identification of Candida spp. by phenotypic tests and PCR. Braz J Microbiol 2010, 41 (2), 286- 94.
- Silva S, Candida glabrata, Candida parapsilosis and Candida tropicalis: biology, epidemiology, pathogenicity and antifungal resistance. FEMS Microbiol Rev 2012, 36 (2), 288- 305.
- Amaha M, Sai T. Some aspects of (-)-citramalic acid accumulation by respiration-deficient mutants of yeasts. Antonie Van Leeuwenhoek. 1969, 35, Suppl:G15-6.
- King N. Mitochondria the Dynamic Organelle. In Amino Acids adn the mitochondria, SW, S.; MS, S., Eds. Springer: New York, NY, 2007; pp 151-166.
- El Golli Bennour E, et al. Toxicities induced in cultured human hepatocarcinoma cells exposed to ochratoxin A: oxidative stress and apoptosis status. J Biochem Mol Toxicol. 2009, 23 (2), 87-96.
- Bin-Umer MA, et al. Elimination of damaged mitochondria through mitophagy reduces mitochondrial oxidative stress and increases tolerance to trichothecenes. Proc Natl Acad Sci USA. 2014, 111 (32), 11798-803.
- Darif Y, et al. Ochratoxin A mediates MAPK activation, modulates IL-2 and TNF-alpha mRNA expression and induces apoptosis by mitochondria-dependent and mitochondria-independent pathways in human H9 T cells. J Toxicol Sci 2016, 41 (3), 403-16.
- Pace JG, Watts MR., Canterbury WJ. T-2 mycotoxin inhibits mitochondrial protein synthesis. Toxicon 1988, 26 (1), 77-85.
- Assaf H, Azouri H, Pallardy M. Ochratoxin A induces apoptosis in human lymphocytes through down regulation of Bcl-xL. Toxicol Sci 2004, 79 (2), 335-44.
- Pell VR, et al. Succinate metabolism: a new therapeutic target for myocardial reperfusion injury. Cardiovasc Res 2016, 111 (2), 134-41.
- Lalani SR, et al. Isolated mitochondrial myopathy associated with muscle coenzyme Q10 deficiency. Arch Neurol. 2005, 62 (2), 317-20.
- Deponte,M. The Incomplete Glutathione Puzzle: Just Guessing at Numbers and Figures? Antioxid Redox Signa.l 2017, 27 (15), 1130-1161.
- Guilford FT, Hope J. Deficient glutathione in the pathophysiology of mycotoxin-related illness. Toxins (Basel) 2014, 6 (2), 608-23.
- 37.Crawford DR, et al. Characterization of liver injury, oval cell proliferation and cholangiocarcinogenesis in glutathione S-transferase A3 knockout mice. Carcinogenesis. 2017, 38 (7), 717-727.
- Yu YM, et al. Plasma L-5-oxoproline kinetics and whole blood glutathione synthesis rates in severely burned adult humans. Am J Physiol Endocrinol Metab. 2002, 282 (2), E247-58.
- De Santis B, et al. Study on the Association among Mycotoxins and other Variables in Children with Autism. Toxins (Basel) 2017, 9 (7).
- Schrickx JM, et al. Organic acid production by Aspergillus niger in recycling culture analyzed by capillary electrophoresis. Anal Biochem 1995, 231 (1), 175-81.
- Binder RE, et al. Chronic necrotizing pulmonary aspergillosis: a discrete clinical entity. Medicine (Baltimore) 1982, 61 (2), 109-24.
- Al-Jishi E, et al. Clinical, biochemical, and molecular characterization of patients with glutathione synthetase deficiency. Clin Genet 1999, 55 (6), 444-9.
- Shaw W, Kassen E, Chaves E. Assessment of antifungal drug therapy in autism by measurement of suspected microbial metabolites in urine with gas chromatography-mass spectrometry. Clinical Practice of Alternative Medicine. 2000, 15-26.
- Danpure C. Primary Hyperoxaluria. In The Online Metabolic and Molecular Bases of Inherited Disease, The McGraw Hill Companies: 2001; p 3326.
- Tanaka K, et al. Gas-chromatographic method of analysis for urinary organic acids. II. Description of the procedure, and its application to diagnosis of patients with organic acidurias. Clin Chem. 1980, 26 (13), 1847-53.